ABSTRACT
The traditional farming system managed to preserve the soil health and remained resilient for crop production. Introduction of modern agriculture practice, the Green Revolution in 1970s had increased the farm productivity and managed to reduce famine in Asia. Unfortunately, implementation of this new agriculture practice caused various adverse effects in the long period; the soil health was degraded in many parts of the world. Soil health degradation caused the declining productivity which became food insecurity issues for increasing population. On the other hand, this contributed to various environmental issues and also contributed to global warming. In this article, the factors that influence the soil health degradation in Asia were discussed. Principles of soil health and the strategies for soil health restoration were also discussed to give insight on how to increase agricultural resilience which will directly contribute to food security. Rehabilitation of arid soil such as desert and sand tailing will give a new hope for crop production and food security. Restoration of soil health is also very crucial to tackle various environmental issues such as pollution, man-induced disasters such as flood caused by deforestation, emission of greenhouse gas and climate change.
Keywords: soil health, resilience, soil degradation, soil restoration, food security
INTRODUCTION
Farming, which took root 12,000 years ago, triggered changes in society from a traditional hunter-gatherer lifestyle to a permanent settlement and a reliable food supply. Although the land was used for agriculture, the soil quality remains undisturbed and very healthy. Since then, the soil became medium for food production and supplied nutrients for human beings. This healthy soil managed to protect itself from various natural calamities and remained resilient for agriculture and food production. Soil health can be preserved by implementing six basic principles: i) limited disturbance to soil; ii) maximized armour/soil cover; iii) maximized diversity; iv) maximized living roots; v) plant-animal integration; and vi) crop cultural practices.
Soil health is defined as the continued capacity of soil to function as a vital living ecosystem that sustains plants, animals and humans (USDA-NRCS, 2019). A lengthier version of definition as suggested by Doran and Zeiss (2000) is “the capacity of soil to function as a vital living system, within ecosystem and land use boundaries, to sustain plant and animal productivity, maintain or enhanced water and air quality, and promote plant and animal health”. The characteristics of healthy soils are possessed good soil tilth, sufficient depth, good water storage and drainage, sufficient supply, but not excess of nutrients, small population of plant pathogens and insect pests, large population of beneficial organisms, low weed pressure, free of chemicals and toxins that harm the crop, resistant to degradation and resilience when unfavorable condition occur (Moebius-Clune et al, 2016).
Soil resilience is the intrinsic ability of the soil to recover from degradation and return to a new equilibrium similar to the antecedent state. Soil resilience is also referred as ability of the soil to recover its “functional and structural integrity (Seybold et al, 1999). Soil resilience is important for the soil to restore its health and fertility and therefore continuous farming with satisfactory productivity can be maintained. However, if the degradation of the soil exceeds the threshold level, the soil will lose it’s resilience and contribute to its health degradation as well (Blanco-Canqui and Lal, 2010). However, the introduction of Green Revolution had significantly contributed to good impacts for human beings and bad impacts for soil if the implementation of this agricultural practices were mismanaged.
The Green Revolution which began in the 1970s changed the whole scenario of agriculture in the globe. Crop production was intensified through high-yielding varieties, irrigation systems, usage of synthetic chemical fertilizers, pesticides for pest and disease control, intensive tillage and mono-culture farming (Setboonsang and Gregorio, 2017). This modern agricultural system increased yields significantly and contributed to poverty reduction in many parts of the world, particularly in Asia although in Africa it was less successful and famine was not abolished there. However, the adversaries of this modern agricultural practices on humans and the environment have become more evident and could not be neglected (Setboonsang and Gregorio, 2017). Soil health deterioration, losses of soil resilience, contamination of water bodies, losses of biodiversity and global warming were reported related to intensive agriculture as a result of the Green Revolution.
FAO had estimated that 1/3 of world’s soil was degraded, indicating less arable soil for crops production for the increasing world population (FAO, 2015). World Resource Institute estimated rise in global food demand as much as 50% by 2050 to feed the increasing world’s population. However, this soil health degradation will jeopardize the aim to increase food by 50% and possibly threaten the food security in many parts of the world then.
SOIL HEALTH DEGRADATION
Continuous practice of intensive agriculture as a results of Green Revolution implementation for decades which resulted with various undesirable impacts on soil health and its resilience. FAO estimated that 1/3 of world’s soil was degraded and possess risk of less arable soil for crop production (Earth Worm Foundation, 2022). Soil degradation is defined as a change in the soil health status resulting in a diminished capacity of the ecosystem to provide goods and services for its beneficiaries. Degraded soils have a health status such that they do not provide the normal goods and services of the particular soil in its ecosystem (FAO, 2022). Among the soil degradation threats reported related to this intensive agriculture practices are as listed below:
Erosion
Soil erosion is the most important threat to soil in Asia mainly in South and East Asia. In the drier regions or deserts, wind was the main driving force that induces erosion. Water erosion mainly caused by surface water flow in humid regions with torrential rains. Climate change also contributed to excessive rain precipitation which speeds up the erosion process. Twenty-one percent of the total land in Asia were degraded by water erosion. China recorded more than > 180 million ha soil degradation caused by water erosion and followed by India (> 90 million ha), Indochina (> 40 million ha), Philippines (10 million ha) and Indonesia (22.5 million ha) (Table 1). Nine per cent of total area in Asia were eroded by winds mainly concentrated in western and northern semi-arid regions such as Pakistan (> 11 million ha), India (> 23.6 million ha) and China (> 78.5 million ha) (Table 1) (Setboonsarng and Gregorio, 2017).
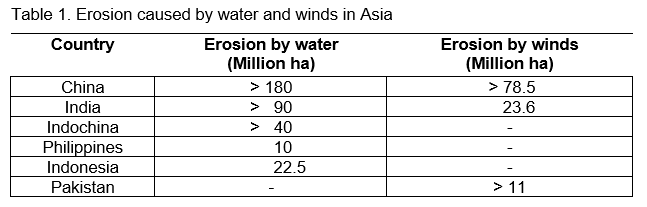
Soil organic carbon loss
Soil organic matter (SOM) in South Asia shows a decreasing pattern in soil because most of the plant residues were used as fuel or fodder for animal, which are not returned to the soil, therefore the SOM in soil in this area is depleted. In Indonesia, three anthropogenic activities, deforestation, poor land management and intensive cropping shown changes in SOM composition in both mineral and peat soils. Failure to cover the soil during land clearing for plantation crop cultivation mainly in South East Asia also contributed to SOM losses especially through water erosion. Heavy rains and frequent tropical storms caused more organic matter losses (Setboonsarng and Gregorio, 2017).
Soil contamination
The arable soil in Asia was easily contaminated with the mining activities, smelting, agrochemical and sewage application and livestock and manure uses. There was an alarming situation of accumulation of hazardous chemicals such as Cd, As, Pb, Cu and Zn in paddy field in various Asian countries such as Bangladesh, India, China, Vietnam, Taiwan, Thailand and Nepal. The hazardous compounds were adsorbed by the plant and accumulated into grains which become a health threat for humans (Setboonsarng and Gregorio, 2017).
Soil acidification
Main parts of soil acidification occur in Southeast Asia and South Asia. Mainly contributed by acid sulphate soil and imbalance fertilizer application of ammonium-based fertilizers. Total area of acid sulphate soil in Southeast Asia is 7.5 million ha. In Vietnam, the increase in fertilizer application and unbalanced use lead to inadequate presentation of acidic factors in soil solution. Application of certain types of organic fertilizers and acidifying fertilizers like ammonium sulphate contributed to soil acidification increase (Setboonsarng and Gregorio, 2017).
Soil salinization and sodification
The salt-affected soils which are widely distributed in the semi-arid and arid zones in the Central and West Asia. Salt-affected soils also showed increasing trend in the coastal area in the South and Southeast Asia that are mainly contributed by intrusion of marine salt water. Increasing salinization in the coastal area could become serious problem for lowland rice production (Setboonsarng and Gregorio, 2017).
Loss of soil biodiversity
Land clearing especially for mono-crop cultivation had significantly reduced the diversity and population of micro and macrofauna. Forest and land clearing for big size plantations for oil palm, rubber and durian showed decreased diversity of micro and macrofauna compared to undisturbed soil. Diversity loss is notable in large scale plantations mainly which contributed to continuous monoculture cropping, over cultivation, intensive use of chemical fertilizers and pesticides, chemical fumigation to prevent soil-born diseases (vegetable production), soil contamination and non-target effects of chemical pesticides and over-application of herbicides. The diversity loss, especially the beneficial organisms, create a conducive environment for disease infections, insect pest infestations and other cropping issues (Setboonsarng and Gregorio, 2017).
Nutrient imbalance
Harvested crops remove nutrients such as N, P, K and other nutrients from agricultural soil, so to sustain the agricultural productivity that requires replacement of nutrients through biological, organic or inorganic sources. Balanced nutrient availability in soil is essential for achieving high value crops, but excessive or imbalanced nutrients composition may pose risks to the environment, human health and ecosystems. When a N fertilizer is first applied to soil, a high response is frequently obtained from nitrogen. Nutrient additions in some Asian countries are excessive which degrade both air and water quality (Setboonsarng and Gregorio, 2017).
Compaction and softening of hardpan
Intensive application of heavy machineries for cultivation and harvest and continuous chemical input application contributed to soil compaction. Highly compacted soil will be deteriorated where decreases in soil porosity occur that affects water content, hydraulic conductivity and gas permeability. Usage of mechanization in soil on wheat cultivation area in India showed increase in compactness of surface soils as indicated by increase in bulk density as high as 1.80 g cm-3. Similar pattern in soil compactness in China was also observed when mechanization was used for plantation forests (Setboonsarng and Gregorio, 2017). Intensive use of heavy machineries in rice fields caused soil softening which disintegrate the hardpan. In 2013, 13,000 ha of rice field in Malaysia were affected with soil softening and hardpan degradation which contribute to decrease in productivity in those affected areas (Taufik et al, 2021).
PRINCIPLES OF SOIL HEALTH
Soil health is defined as capacity of soil to function as vital living ecosystem that sustains plants, animal and humans (USDA, 2019. Healthy soils generated clean air and water, crops and forests, grazing lands and diverse biodiversity (USDA 2019). Soil does all these by performing five essential functions as listed below.
- Regulating water - Soil helps control where rain, snowmelt and irrigation water goes. Water flows over the land or into and through the soil.
- Sustaining plant and animal life - The diversity and productivity of living things depends on soil.
- Filtering and buffering potential pollutants – The minerals and microbes in soil are responsible for filtering, buffering, degrading, immobilizing, and detoxifying organic and inorganic materials, including industrial and municipal by-products and atmospheric deposits.
- Cycling nutrients – Carbon, nitrogen, phosphorus, and many other nutrients are stored, transformed and cycled in the soil.
- Providing physical stability and support – Soil structure provides a medium for plant roots. Soil also provides support for human structures and protection for archaeological treasures.
To achieve all the functions mentioned above, robust strategies need to be devised to improve the soil’s resilience and health thus farm productivity can be elevated as well as sufficient food generation to help achieve global food security.
STRATEGIES FOR SOIL HEALTH RESTORATION FOR RESILIENCE AGRICULTURE AND FOOD SECURITY
Intensive agriculture and improper land use had led to soil health degradation and soil function losses. Restoration of degraded soil is important to make it fit for agriculture thus the soil becomes resilient and fertility will increase as well. Restored degraded soil in Asia will increase the arable land for agriculture that enhances productivity and food security. Effective soil restoration, followed by management strategies are necessary to maintain and enhance the soil health of agricultural land (Guo, 2021). Basically, there are many principles that can be adopted to restore degraded soil as explained below.
Limited disturbance to soil
Soil disturbances were minimized to reduce the introduction of mechanical, chemical and biological disturbances to soil. Crop productivity will be affected when the soil health was disturbed. Besides that, various catastrophises such as floods, mud-floods and loss of habitats will happen if soil health is disturbed. Limiting the soil disturbance will increase productivity and conserve biodiversity and environment.
Zero or limited tillage
Continuous tillage has been found to be averse to soil health. Tillage is one of the common agricultural practices that destroys the soil structure. Tillage also destroys the habitat of beneficial soil fauna such as earth worm and insects. The bad impact of tillage can be lessened by using zero tillage or limited tillage cultivation technique. Zero tillage or limited tillage maintains a permanent or semi-permanent soil cover that protects the soil from sun, rain and wind and allows the soil microorganisms and fauna to take on the task of “tilling” and soil nutrient balancing, a natural process which are disturbed by mechanical tillage. In Indo-Gangetic region, rice followed by wheat cultivation system found that the zero-tillage or minimal tillage is more beneficial by reducing the crop duration, reduction in cost for inputs, maintenance of soil moisture, increase of soil organic matter, enhancement of carbon sequestration, prevention of soil erosion, avoidance of soil compaction caused by heavy machineries and preservation of the soil biodiversity. In India, zero tillage reduces cost of production by nearly US$35-40/ha mainly through cost saving for land preparation and fuel consumption by 50-60 litres of diesel. Prevention of tillage in the crop production also contributed to enhancement of soil organic matter accumulation and reduces water requirement for wheat production in rice-wheat alternate cultivation system (Tumma and Poorna Chandrika, 2022).
Reduce usage of machineries
Continuous usage of machineries in agriculture could cause soil compaction and soften the soil in wet rice fields. Both the soil compaction and soil softening could be managed by using light weight machineries and farm implements. Rice soil softening caused by degradation of hardpan layer is required in paddy fields to support the weight of field machineries for mobility. Replacement of heavy weight machineries with light weight for ploughing, fertilizer and pesticide application and crop harvesting will minimize both the compaction and softening issues of soils (Taufik et al, 2021).
Replenishment of depleted soil organic matter (SOM)
The soil organic matter depletion can be addressed by building up of soil organic carbon. Application of animal manures, cultivation of legume based green manure, tree-leaf based green manuring, crop rotation, inclusion of legumes in crop rotation and sheep-goat penning were practiced in South Asia in the semi-arid and arid tropics regions (Srinivasarao et al, 2019). Utilization of biofertilizers, biopesticidse, avoidance of toxic chemical pesticides and enhancement of microbial biodiversity also contributed to the restoration of degraded soil in South Asia (Srinivasarao et al, 2019).
Armor
Armor is a practice to keep soil covered with living plants, crop residues, compost or plastic sheets. Soil cover helps control soil erosion, check weeds, mitigate soil temperature fluctuation, reduce soil compaction, and provide improved habitats to soil organisms. Plant residues and silver shine (plastic sheet) were the popular soil cover methods in Asian countries. Protected structure such as greenhouses and plastic-netted structures used for crop production were found to prohibit erosion. While living plants such as Mucuna bracteate is popular as life mulch in Malaysia and Indonesia in plantation sectors. In highlands especially wild plants such liverwort and moss played an important role in mitigating soil erosion. Compost also was used as mulching material and have a role in reducing erosion. Soil armor contributed in reducing evaporation rate which helps maintain the moisture in the soil. Covered soil is also resistant against controlling water and wind erosion and reduces soil compaction. Another function of soil armor is to supress weed emergence and growth; therefore, application of weedicide can be minimized to protect the soil and soil biota including microorganisms, invertebrate and other small animals, thus the soil microfauna diversity thrived. Implementation of armor is important to protect habitat on the soil and resist the organic matter losses.
Diversity
Soil biodiversity reflects diverse range of living organisms ranging from invisible microorganisms, meso-fauna and macro fauna within a soil. Plant roots could also be considered as soil organisms because of their symbiotic relations and interactions with other soil components. Soil organisms play important roles in nutrient cycling, regulate the dynamics of soil organic matter, soil carbon sequestration and greenhouse gas emission, modifying soil’s physical structure and water regimes, improve the nutrient use efficiency and enhance plant health (FAO, 2022). Increasing soil biodiversity contributed to soil resilience enhancement against environmental challenges.
Soil health can be promoted by increasing the soil biodiversity. Application of organic input, reduction of inorganic input application, and reduction of tillage act together to promote biological activity which resulted in increased resilience in extreme events. Cultivation of multi-crops instead of mono-crop could enhance the soil biodiversity and improve soil health. Cultivation of cover-crop and mulching also contributed to soil biodiversity. Cultivation of host crop, such as Prosopis juliflora L., assisted the nutrients absorption for sandalwood (Santalum album L.). The sandalwood-Prosopis combination has been found to improve the vegetative growth and physiological functions of sandalwood (Srikantaprasad et al, 2022). Combination of all the factors mentioned above can improve soil biodiversity and enhance plant health that contribute to soil resilience and increase productivity (SRDP Farm Advisory Service, 2019).
Living roots
Roots play important roles not only for the plant’s physical anchoring on the soil, but also contribute to soil health. Roots provide structure for soil to stick on during adverse conditions such as heavy winds or torrential rains, thus risk of erosion and flash floods can be averted. Roots also help in removing soil particles from its surroundings and made soil less compacted and more porous. Roots also percolate water until the underground water source. Roots also behave as intermediate for nutrient exchange from soil to plant and carbon (carbohydrate) from plant to soil. Roots excreted 10-40% of the photosynthate in the form of exudates that mainly contains carbohydrates, amino acids, organic acids, proteins, sugar, phenolic compounds and other substances. The exudates have many functions such as solubilizing nutrients such as phosphate or potassium from soil particles, change the redox state of the root surface chelate iron and zinc, and desorb nutrients from clay surfaces. Microorganisms such as bacteria and fungus feed on the sugars excreted by exudates thus can thrive in the soil and contribute to soil health. Soil microorganisms such as rhizobia and arbuscular mycorrhizal fixes atmospheric nitrogen for plant consumption. Thriving of indigenous beneficial microorganisms such as biofertilizers and biopesticides strains could offset the negative effects of pathogens through assisting plants in defence against pathogens and diseases. Optimal population of indigenous biopesticide strains make the soil suppressive against pathogens, thus lessen the usage of chemical pesticides that indirectly contributed to soil health. Plant roots complete a cycle in the soil. The deeper the root penetrates; they provide more benefits to soil fertility and carbon storage in the soil. When alive, roots redistribute carbon and nutrients throughout the soil profile. When roots die, it decays and leaves more organic matter which contribute to more stable and longer lasting soil organic carbon.
Plant-animal integration
Plant-animal integration system is an approach where livestock was raised together with crops in farms. Various integrated systems such as sod-based farming, cover crop as forage, ley farming, pasture cropping, dual purpose cereal crops and agroforestry and alley cropping are suitable for integrated crops-animal farming (Lal, 2020). The residue generated from the crop production can be used as fodder for animals, while the manure and animal urine generated can be applied as organic fertilizer for crop production. The animal manure could improve the organic matters level in the soil. This plant-animal integration is efficient in carbon sequestration and reduces the enteric gas emission.
Crop cultural practices
Various environmentally friendly crop production practices in place as improvement of the original green revolution practices. Conservation agriculture, biological agriculture, natural sequencing farming, integrated nutrient management (INM), site-specific nutrient management (SSNM) and circular farming among the agricultural practices that reduces the bad impact of continuous implementation of conventional farming.
Conservation farming
Conservation farming is an approach in which soil management practices are reduced to a minimum, thus preserving the soil properties and nature’s biodiversity. Implementation of this practice contributed to the conservation of soil, water and soil moisture. It enhances fertilizer and seed uses which saves time and money. Main principles of this approach are minimum soil disturbance, crop residue management (leaving previous crop residue in the field or planting cover crops) and crop rotation practices. The soil is tilled just enough to be possible to sow seeds and leave at least 30% of soil surface covered by crop residue or cover crop (Agrivi, 2022).
Biological farming
Biological farming is a system that works closely with nature which balances the soil to produce healthy, pests and disease resistant crops while reducing the use of chemicals. The basic principles of the biological farm are balanced application of nutrients, fertilizers that possess less damage to soil life and plant roots, responsible application of pesticides and herbicides, create maximum plant diversity by using green manure and crop rotation, maximize decay of organic matters and supply the soil with carbon from compost, green manures, livestock manures and crop residues. This practical system offers a sustainable and profitable agriculture practice that also promotes the soil health (Midwestern BIOaG, 2022).
Circular agriculture
Circular agriculture focuses on the use of minimal amounts of external inputs, closing nutrient loops, regenerating soil and minimizing the impact on the environment. Circular agriculture aims to address the rising concerns about the unsustainability of global food production, soil destruction, loss of biodiversity and depletion, degradation and pollution of water and land resources. Circular agriculture supports a food system from growing to harvesting, packing, processing, transporting, marketing, consuming and disposing of food that are designed with a view of sustainable development (United Nations, 2021). Circular agriculture encourages mixed crop-livestock and organic farming, agroforestry and water recycling and wastewater reuse which are aimed to reduce CO2 emissions, more efficient utilization of natural resources and minimize the use of input significantly. As an example, circular agriculture can be employed by combining crop cultivation with animal husbandry where the wastes generated from the crop cultivation either can be used as fodder for animal or composted as organic fertilizer. The manure and urine generated from animal husbandry can be used as organic fertilizer or can be used as amendments for crop residues composting. If the farm has fish ponds, the water from the pond can be used to irrigate the crops, in return the crop residues can be used as fodder for the fish. Agroforestry is another strategy which combines tree planting with crops or pastures. The pasture can be used for farm animal grazing. Recycling and reuse of irrigation is an important part of circular agriculture water management which addresses the water scarcity issues.
Circular agriculture is more sustainable with less inputs as opposed to the big scale monoculture farming. However, this circular agriculture is labor intensive, therefore it is not suitable for big-scale farms. This system is suitable for smaller scale farms mainly for food production which contributes to food security in the rural areas and poor countries. This system promises resilient agriculture that contributes to soil health improvement.
Organic farming
Organic farming is an agricultural system which uses ecological approach in pests and diseases control and biologically derived fertilizers mainly from animal and plant wastes and nitrogen fixing cover crops. Modern organic farming does not use chemical pesticides, reduces soil erosion, decreases nitrate leaching into groundwater and surface water, and recycles back animal wastes into farms. The soil organic matter level will increase and the soil will become healthier with continuous organic farming. Circular agriculture is best suited for organic farming provided that organic inputs and pest and disease control measures are applied.
Integrated Nutrient Management (INM)
Integrated Nutrient Management (INM) is an approach that manages both organic and inorganic plant nutrients for optimal production of cultivated crops, forage and tree species, while conserving the natural resource base that is essential for long term sustainability (Smalling, 1993). Organic nutrient inputs can be obtained from plant residues such as rice straw or Plant Growth Promoting Rhizobacteria (PGPR). Compost generated from crop residues is a nutrient rich organic fertilizer that can be easily produced at the farm level at a very low cost. PGPR is defined as a heterogeneous group of non-pathogenic free-living bacteria that are associated with plant roots and mediate improvements in plant growth and/or health (Kloepper et al., 1989; Bent, 2006). The PGPR contributes by providing nutrients for plant growth including atmospheric N2 fixation, releasing of P from soil and organic matters, chelates ferric ion from soil, improves the plant root system, and reduces biotic and abiotic stress which can decrease the plant’s vigor and health. The combined usage of organic fertilizers, decreased amounts of chemical inputs, and the addition of biofertilizers could significantly improve nutrient management in crop production and reduces the dependence on imported chemical fertilizers. Based on our own experience, the application of 50% of recommended rate of chemical fertilizers and biofertilizer combination managed to produce yield as good as 100% of the recommended rate of chemical fertilizer alone. Based on 3 years of extensive participatory observation in the Mekong Delta showed reduction in fertilizer needs as high as 52% in rice production (Nguyen et al, 2017). This would in turn reduce the spending in the purchase of chemical fertilizers thus saving on production cost. In addition, this INM approach can also promote an environmentally friendly and sustainable agriculture practice by reducing environmental contamination. As an added benefit, the usage of PGPR also helps the environment by reducing greenhouse gas emissions from agriculture.
Site Specific Nutrient Management (SSNM)
Fertilizer application recommendation are often determined based on crop response data averaged over large area although farmer’s fields show large variability in terms of nutrient supplying capacity and crops response to nutrients (Richard et al, 2022). Thus, blanket application of fertilizer may lead to over-fertilization in some areas and under-fertilization in others, or the application of an improper balance of nutrients for the soil or crops. As an alternative for blanket fertilizer application, Site Specific Nutrient Management (SSNM) aims to optimize the supply of soil nutrients over time and space to match the requirement of crops through four principles namely right product, right rate, right time and right place (Richard et al, 2022). The selected fertilizer products such as commercial fertilizers, livestock manure, compost and crop residue must ensure balanced supply of nutrients and suits the soil properties. The rate of fertilizer application is determined by the fertility status of sites determined through soil test. The optimal time for fertilizer application is determined based on nutrient requirement for different growth stages. The application place for fertilizer such as placing and keeping nutrients at optimal distance from the crop and soil depth could minimize the nutrient losses. The amount of nutrients will be varied based on the nutrient availability in the soil. Based on Witt and Dawe (2002) experience, the productivity of rice in SSNM plot can be continuously increased to 4 seasons with efficient nutrient application. In Malaysia, implementation of SSNM with optimum nutrient application determined by using RiceFert modelling system had increased the rice production (DOA, 2019).
Rehabilitation of arid soil that is unfit for agriculture
The size of desert in Asia amounted 3.83 million square km which covers the Arab Peninsula, Gulf countries, Iran, Iraq, China, India, Mongolia and Pakistan. These arid soil structures are very poor and receive very little rain making it unsuitable for crop production. However, latest invention by using clay and glue had improved the sand structure that makes it suitable for crop production. United Arab Emirates, Saudi Arabia and China were among the countries which had adopted this technology to rehabilitate their desert and crop production. In Malaysia, tin mining had produced sand tailing which is totally unfit for crop production. However, this unfit soil can be rehabilitated by burying empty fruit bunch or compost, which made it fit for cultivation of vegetables, tubers and small size fruit trees. Rehabilitation of deserts and sand tailings offer more land area for crop production which will generate extra food and contribute to food security. These rehabilitation technologies bring a new ray of hope for food security.
CONCLUSION
Intensive conventional agriculture practices had caused many adverse effects that directly deteriorate the soil health, decrease soil fertility and created environmental issues. Restoration of this degraded soil is urgently needed for crop production and fulfil food demand of an increasing population. Various, strategies are available and proven working for the restoration of degraded soil. It is still not too late to rehabilitate this unhealthy soil and turn it as productive crop farms. Failure to react now in order to restore the degraded soil will push to an irreversible state of degraded soil. Rehabilitation of soil that is totally unfit for crop production such as desert and sand tailing shed a new ray of hope for global food security and humanity.
REFERENCES
Agrivi. 2022. How can conservation farming reduce climate change? Preprint at https://www.agrivi.com/blog/how-can-conservation-farming-reduce-climate-change/ 2022/07/25.
Blanco-Canqui, H. and Lal, R. 2010. Soil resilience and conservation. P. 425-447. In Principles of Soil Conservation and Management, 425-447, Springer, Dordrecht. https://doi.org/10.1007/978-1-4020-8709-7_16
Doran, J.W.,and Zeiss, M.R..2000. Soil health and sustainability: Managing the biotic component of soil quality. Applied Soil and Ecology, 15, 3-11.
DOA. 2019. Pengurusan Nutrient Lokasi Spesifik: Jelapang Padi Pulau Pinang.pp 172, DOA Printing, Putrajaya.
Earth Worm Foundation. 2022. Look Down Earth. Preprint at https://www.earthworm.org/campaigns/lookdowntoo?gclid=CjwKCAjwwo-WBhAMEiwAV4dybdT2f_3If_ZVId6fxulGlaIYQ39faJ_1Jb6PFDYi8po-yWZRFYcuMRoC-WEQAvD_BwE/ 2022/06/30
FAO. 2015. Status of World’s Soil Resources – Main Report. Chapter 10: Regional Assessment of Soil Change in Asia. FAO, Rome, Italy, pp. 329.
FAO. 2022. FAO Soil Portal. Preprint at https://www.fao.org/soils-portal/soil-biodiversity/en/ 2022/07/14
Guo, M. 2021. Soil health assessment and management: Recent development in science and practices. Soil System 5, 61.
Kloepper, J.W., Lifshitz, R. and Zablotowicz, R.M. 1989. Free-living bacterial inocula for enhancing crop productivity. Trends in Biotechnology 7, 39-44.
Lal, R. 2020. Integrating animal husbandry with crops and trees. Frontiers in Sustainable Food Systems 4:113 doi: 10.3389/fsufs.2020.00113
Midwestern BIOaG. 2022. About Biological Farming. Preprint at https://www.midwesternbioag.com/about/ about-biological-farming/ 2022/07/25.
Moebius-Clune, B.N., Moebius-Clune, D.J., Gugino, B.K., Idowu, O.J., Schindelbeck, R.R., Ristow, A.J., van ES< H.M., Thies, J.E., Shayler, H.A., McBride, M.B., Kurtz, K.S.M., Wolfe, D.W. and Abawi, G.S. 2016. Comprehensive Assessment of Soil Health – The Cornell Framework, Edition 3.2, Cornell University, Geneva, NY, pp.134.
Nguyen, T.H., Phan, T.C. Choudhury, A.T.M.A, Tose, M.T, Deaker, R.J. and Kennedy, I.R. 2017. BioGro: A Plant Growth Promoting biofertilizer validated by 15 years’research from laboratory selection to rice farmer’s fields of the Mekong Delta. In Agro-Environmental Sustainability, Ed. J.S. Singh and G. Seneviratne (eds), 237-254. DOI 10.1007/978-3-319-49724-2_11
Richards, M.B., Buttetbach-Bahl, K., Jat, M.L., Lipinski, B., Ortiz-Monaterio, I. and Sapkota, T. 2022. Site-Specific Nutrient Management: Implementation guidance for policymaker and investors. Global Alliance for Climate-Smart Agriculture (GASCA), pp.10. Preprint at https://ccafs.cgiar.org/resources/publications/site-specific-nutrient-management-implementation-guidance-policymakers/ 2022/07/26
Setboonsarng, S. & Gregorio, E. 2017. Lage scale soil health restoration: The way forward for reversing climate change while enhancing food and nutrition security. ADB Southeast Asia Working Paper Series, No. 16, pp. 31.
Seybold, C.A., Herrick, J.E. and Bredja, J.J 1999. Soil resilience: A fundamental component of soil quality. Soil Science, 164(4): 224-234.
Smaling, E.M.A. 1993. Soil nutrient in Sub-Saharan Africa. In: The Role of Plant Nutrients for Sustainable Food Crop Production in Sub-Saharan Africa, eds. H. van Reuler and W.H. Prins, pp. 53-67, Leidschendam, the Netherlands: Vereniging van Kunstmest Producenten.
SRDP Farm Advisory Service. 2019. Technical Note 271: Soil biodiversity and soil health.pp. 5. Preprint at https://www.fas.scot/downloads/tn721-soil-biodiversity-and-soil-health/ 2022/07/03
Srikantaprasad, D., Malliarjuna Gowda, A.P., Pushpa, T.N., Thimmegowda, M.N., Umesha, K., Ravikumar, R.I and Prasanna, K.T. 2022. Identification of suitable host for sandalwood cultivation in Northern dry zone of Karnataka. Industrial Crops and Products 182: 114874 (in press)
Srinivasarao, C., Kundu, S., Subha Lakshmi, C., Sudha Rani, Y., Nataraj, K.C., Gangaiah, B., Jaya Laxmi, M., Vijay Sankar Babu, M., Rani, U., Nagalakshmi, S. and Manasa, R. 2019. Soil health issues for sustainability of South Asia Agriculture. EC Agriculture 5.6.
Taufik, A. M., Zaman, O. M. F., Fauzi, M. I. M., Khusairy, K.M., Herman, S.E., Hashim, A.M., Azlan, O., Saifulizan, M.N., Bunyamin, A.K. and Mansor, N. 2021 Effect of mole drain and tracked agricultural prime movers on soft soil paddy areas. Advances in Agricultural and Food Research Journal, 2(2): a0000228. Preprint at https://doi.org/10.36877/aafrj.a000022/2022/07/03.
Triplett Jr., G.B. & Dick, W.A. 2008. No-tillage crop production: Revolution in agriculture. Agronomy Journal 100: S1153 – S-165. Preprint at https://doi.org/10.2134/agronj2007.0005c/ 2022/06/30
Tumma, M.K and Poorna Chandrika, K.S.V. 2022. Zero tillage Zero worries. Vikaspedia. Preprint at https://vikaspedia.in/agriculture/best-practices/sustainable-agriculture/crop-management/201czero-tillage201d-zero-worries/ 2022/07/22
USDA-NRCS (2019). Soil Health; Natural Resource Conservation Service, U.S. Department of Agriculture: Washington DC, USA, 2019. Preprint at https://www.nrcs.usda.gov/wps/portal/nrcs/detail/pa/soils/health/?cid=nrcseprd1200408/ 2022/07/18
United Nation (2021) UN/DESA Policy Brief # 105: Circular agriculture for sustainable rural development. Preprint at https://www.un.org/development/desa/dpad/publication/un-desa-policy-brief-105-circular-agriculture-for-sustainable-rural-development/ 2022/07/22
Witt, C. and Dawe, D. 2014. Performance of Site-Specific Nutrient Management in intensive rice cropping systems of Asia. Better Crops International 16 (1): 25-30.
Healthy Soils for Resilient Agriculture towards Enhancement of Food Security in Asia
ABSTRACT
The traditional farming system managed to preserve the soil health and remained resilient for crop production. Introduction of modern agriculture practice, the Green Revolution in 1970s had increased the farm productivity and managed to reduce famine in Asia. Unfortunately, implementation of this new agriculture practice caused various adverse effects in the long period; the soil health was degraded in many parts of the world. Soil health degradation caused the declining productivity which became food insecurity issues for increasing population. On the other hand, this contributed to various environmental issues and also contributed to global warming. In this article, the factors that influence the soil health degradation in Asia were discussed. Principles of soil health and the strategies for soil health restoration were also discussed to give insight on how to increase agricultural resilience which will directly contribute to food security. Rehabilitation of arid soil such as desert and sand tailing will give a new hope for crop production and food security. Restoration of soil health is also very crucial to tackle various environmental issues such as pollution, man-induced disasters such as flood caused by deforestation, emission of greenhouse gas and climate change.
Keywords: soil health, resilience, soil degradation, soil restoration, food security
INTRODUCTION
Farming, which took root 12,000 years ago, triggered changes in society from a traditional hunter-gatherer lifestyle to a permanent settlement and a reliable food supply. Although the land was used for agriculture, the soil quality remains undisturbed and very healthy. Since then, the soil became medium for food production and supplied nutrients for human beings. This healthy soil managed to protect itself from various natural calamities and remained resilient for agriculture and food production. Soil health can be preserved by implementing six basic principles: i) limited disturbance to soil; ii) maximized armour/soil cover; iii) maximized diversity; iv) maximized living roots; v) plant-animal integration; and vi) crop cultural practices.
Soil health is defined as the continued capacity of soil to function as a vital living ecosystem that sustains plants, animals and humans (USDA-NRCS, 2019). A lengthier version of definition as suggested by Doran and Zeiss (2000) is “the capacity of soil to function as a vital living system, within ecosystem and land use boundaries, to sustain plant and animal productivity, maintain or enhanced water and air quality, and promote plant and animal health”. The characteristics of healthy soils are possessed good soil tilth, sufficient depth, good water storage and drainage, sufficient supply, but not excess of nutrients, small population of plant pathogens and insect pests, large population of beneficial organisms, low weed pressure, free of chemicals and toxins that harm the crop, resistant to degradation and resilience when unfavorable condition occur (Moebius-Clune et al, 2016).
Soil resilience is the intrinsic ability of the soil to recover from degradation and return to a new equilibrium similar to the antecedent state. Soil resilience is also referred as ability of the soil to recover its “functional and structural integrity (Seybold et al, 1999). Soil resilience is important for the soil to restore its health and fertility and therefore continuous farming with satisfactory productivity can be maintained. However, if the degradation of the soil exceeds the threshold level, the soil will lose it’s resilience and contribute to its health degradation as well (Blanco-Canqui and Lal, 2010). However, the introduction of Green Revolution had significantly contributed to good impacts for human beings and bad impacts for soil if the implementation of this agricultural practices were mismanaged.
The Green Revolution which began in the 1970s changed the whole scenario of agriculture in the globe. Crop production was intensified through high-yielding varieties, irrigation systems, usage of synthetic chemical fertilizers, pesticides for pest and disease control, intensive tillage and mono-culture farming (Setboonsang and Gregorio, 2017). This modern agricultural system increased yields significantly and contributed to poverty reduction in many parts of the world, particularly in Asia although in Africa it was less successful and famine was not abolished there. However, the adversaries of this modern agricultural practices on humans and the environment have become more evident and could not be neglected (Setboonsang and Gregorio, 2017). Soil health deterioration, losses of soil resilience, contamination of water bodies, losses of biodiversity and global warming were reported related to intensive agriculture as a result of the Green Revolution.
FAO had estimated that 1/3 of world’s soil was degraded, indicating less arable soil for crops production for the increasing world population (FAO, 2015). World Resource Institute estimated rise in global food demand as much as 50% by 2050 to feed the increasing world’s population. However, this soil health degradation will jeopardize the aim to increase food by 50% and possibly threaten the food security in many parts of the world then.
SOIL HEALTH DEGRADATION
Continuous practice of intensive agriculture as a results of Green Revolution implementation for decades which resulted with various undesirable impacts on soil health and its resilience. FAO estimated that 1/3 of world’s soil was degraded and possess risk of less arable soil for crop production (Earth Worm Foundation, 2022). Soil degradation is defined as a change in the soil health status resulting in a diminished capacity of the ecosystem to provide goods and services for its beneficiaries. Degraded soils have a health status such that they do not provide the normal goods and services of the particular soil in its ecosystem (FAO, 2022). Among the soil degradation threats reported related to this intensive agriculture practices are as listed below:
Erosion
Soil erosion is the most important threat to soil in Asia mainly in South and East Asia. In the drier regions or deserts, wind was the main driving force that induces erosion. Water erosion mainly caused by surface water flow in humid regions with torrential rains. Climate change also contributed to excessive rain precipitation which speeds up the erosion process. Twenty-one percent of the total land in Asia were degraded by water erosion. China recorded more than > 180 million ha soil degradation caused by water erosion and followed by India (> 90 million ha), Indochina (> 40 million ha), Philippines (10 million ha) and Indonesia (22.5 million ha) (Table 1). Nine per cent of total area in Asia were eroded by winds mainly concentrated in western and northern semi-arid regions such as Pakistan (> 11 million ha), India (> 23.6 million ha) and China (> 78.5 million ha) (Table 1) (Setboonsarng and Gregorio, 2017).
Soil organic carbon loss
Soil organic matter (SOM) in South Asia shows a decreasing pattern in soil because most of the plant residues were used as fuel or fodder for animal, which are not returned to the soil, therefore the SOM in soil in this area is depleted. In Indonesia, three anthropogenic activities, deforestation, poor land management and intensive cropping shown changes in SOM composition in both mineral and peat soils. Failure to cover the soil during land clearing for plantation crop cultivation mainly in South East Asia also contributed to SOM losses especially through water erosion. Heavy rains and frequent tropical storms caused more organic matter losses (Setboonsarng and Gregorio, 2017).
Soil contamination
The arable soil in Asia was easily contaminated with the mining activities, smelting, agrochemical and sewage application and livestock and manure uses. There was an alarming situation of accumulation of hazardous chemicals such as Cd, As, Pb, Cu and Zn in paddy field in various Asian countries such as Bangladesh, India, China, Vietnam, Taiwan, Thailand and Nepal. The hazardous compounds were adsorbed by the plant and accumulated into grains which become a health threat for humans (Setboonsarng and Gregorio, 2017).
Soil acidification
Main parts of soil acidification occur in Southeast Asia and South Asia. Mainly contributed by acid sulphate soil and imbalance fertilizer application of ammonium-based fertilizers. Total area of acid sulphate soil in Southeast Asia is 7.5 million ha. In Vietnam, the increase in fertilizer application and unbalanced use lead to inadequate presentation of acidic factors in soil solution. Application of certain types of organic fertilizers and acidifying fertilizers like ammonium sulphate contributed to soil acidification increase (Setboonsarng and Gregorio, 2017).
Soil salinization and sodification
The salt-affected soils which are widely distributed in the semi-arid and arid zones in the Central and West Asia. Salt-affected soils also showed increasing trend in the coastal area in the South and Southeast Asia that are mainly contributed by intrusion of marine salt water. Increasing salinization in the coastal area could become serious problem for lowland rice production (Setboonsarng and Gregorio, 2017).
Loss of soil biodiversity
Land clearing especially for mono-crop cultivation had significantly reduced the diversity and population of micro and macrofauna. Forest and land clearing for big size plantations for oil palm, rubber and durian showed decreased diversity of micro and macrofauna compared to undisturbed soil. Diversity loss is notable in large scale plantations mainly which contributed to continuous monoculture cropping, over cultivation, intensive use of chemical fertilizers and pesticides, chemical fumigation to prevent soil-born diseases (vegetable production), soil contamination and non-target effects of chemical pesticides and over-application of herbicides. The diversity loss, especially the beneficial organisms, create a conducive environment for disease infections, insect pest infestations and other cropping issues (Setboonsarng and Gregorio, 2017).
Nutrient imbalance
Harvested crops remove nutrients such as N, P, K and other nutrients from agricultural soil, so to sustain the agricultural productivity that requires replacement of nutrients through biological, organic or inorganic sources. Balanced nutrient availability in soil is essential for achieving high value crops, but excessive or imbalanced nutrients composition may pose risks to the environment, human health and ecosystems. When a N fertilizer is first applied to soil, a high response is frequently obtained from nitrogen. Nutrient additions in some Asian countries are excessive which degrade both air and water quality (Setboonsarng and Gregorio, 2017).
Compaction and softening of hardpan
Intensive application of heavy machineries for cultivation and harvest and continuous chemical input application contributed to soil compaction. Highly compacted soil will be deteriorated where decreases in soil porosity occur that affects water content, hydraulic conductivity and gas permeability. Usage of mechanization in soil on wheat cultivation area in India showed increase in compactness of surface soils as indicated by increase in bulk density as high as 1.80 g cm-3. Similar pattern in soil compactness in China was also observed when mechanization was used for plantation forests (Setboonsarng and Gregorio, 2017). Intensive use of heavy machineries in rice fields caused soil softening which disintegrate the hardpan. In 2013, 13,000 ha of rice field in Malaysia were affected with soil softening and hardpan degradation which contribute to decrease in productivity in those affected areas (Taufik et al, 2021).
PRINCIPLES OF SOIL HEALTH
Soil health is defined as capacity of soil to function as vital living ecosystem that sustains plants, animal and humans (USDA, 2019. Healthy soils generated clean air and water, crops and forests, grazing lands and diverse biodiversity (USDA 2019). Soil does all these by performing five essential functions as listed below.
To achieve all the functions mentioned above, robust strategies need to be devised to improve the soil’s resilience and health thus farm productivity can be elevated as well as sufficient food generation to help achieve global food security.
STRATEGIES FOR SOIL HEALTH RESTORATION FOR RESILIENCE AGRICULTURE AND FOOD SECURITY
Intensive agriculture and improper land use had led to soil health degradation and soil function losses. Restoration of degraded soil is important to make it fit for agriculture thus the soil becomes resilient and fertility will increase as well. Restored degraded soil in Asia will increase the arable land for agriculture that enhances productivity and food security. Effective soil restoration, followed by management strategies are necessary to maintain and enhance the soil health of agricultural land (Guo, 2021). Basically, there are many principles that can be adopted to restore degraded soil as explained below.
Limited disturbance to soil
Soil disturbances were minimized to reduce the introduction of mechanical, chemical and biological disturbances to soil. Crop productivity will be affected when the soil health was disturbed. Besides that, various catastrophises such as floods, mud-floods and loss of habitats will happen if soil health is disturbed. Limiting the soil disturbance will increase productivity and conserve biodiversity and environment.
Zero or limited tillage
Continuous tillage has been found to be averse to soil health. Tillage is one of the common agricultural practices that destroys the soil structure. Tillage also destroys the habitat of beneficial soil fauna such as earth worm and insects. The bad impact of tillage can be lessened by using zero tillage or limited tillage cultivation technique. Zero tillage or limited tillage maintains a permanent or semi-permanent soil cover that protects the soil from sun, rain and wind and allows the soil microorganisms and fauna to take on the task of “tilling” and soil nutrient balancing, a natural process which are disturbed by mechanical tillage. In Indo-Gangetic region, rice followed by wheat cultivation system found that the zero-tillage or minimal tillage is more beneficial by reducing the crop duration, reduction in cost for inputs, maintenance of soil moisture, increase of soil organic matter, enhancement of carbon sequestration, prevention of soil erosion, avoidance of soil compaction caused by heavy machineries and preservation of the soil biodiversity. In India, zero tillage reduces cost of production by nearly US$35-40/ha mainly through cost saving for land preparation and fuel consumption by 50-60 litres of diesel. Prevention of tillage in the crop production also contributed to enhancement of soil organic matter accumulation and reduces water requirement for wheat production in rice-wheat alternate cultivation system (Tumma and Poorna Chandrika, 2022).
Reduce usage of machineries
Continuous usage of machineries in agriculture could cause soil compaction and soften the soil in wet rice fields. Both the soil compaction and soil softening could be managed by using light weight machineries and farm implements. Rice soil softening caused by degradation of hardpan layer is required in paddy fields to support the weight of field machineries for mobility. Replacement of heavy weight machineries with light weight for ploughing, fertilizer and pesticide application and crop harvesting will minimize both the compaction and softening issues of soils (Taufik et al, 2021).
Replenishment of depleted soil organic matter (SOM)
The soil organic matter depletion can be addressed by building up of soil organic carbon. Application of animal manures, cultivation of legume based green manure, tree-leaf based green manuring, crop rotation, inclusion of legumes in crop rotation and sheep-goat penning were practiced in South Asia in the semi-arid and arid tropics regions (Srinivasarao et al, 2019). Utilization of biofertilizers, biopesticidse, avoidance of toxic chemical pesticides and enhancement of microbial biodiversity also contributed to the restoration of degraded soil in South Asia (Srinivasarao et al, 2019).
Armor
Armor is a practice to keep soil covered with living plants, crop residues, compost or plastic sheets. Soil cover helps control soil erosion, check weeds, mitigate soil temperature fluctuation, reduce soil compaction, and provide improved habitats to soil organisms. Plant residues and silver shine (plastic sheet) were the popular soil cover methods in Asian countries. Protected structure such as greenhouses and plastic-netted structures used for crop production were found to prohibit erosion. While living plants such as Mucuna bracteate is popular as life mulch in Malaysia and Indonesia in plantation sectors. In highlands especially wild plants such liverwort and moss played an important role in mitigating soil erosion. Compost also was used as mulching material and have a role in reducing erosion. Soil armor contributed in reducing evaporation rate which helps maintain the moisture in the soil. Covered soil is also resistant against controlling water and wind erosion and reduces soil compaction. Another function of soil armor is to supress weed emergence and growth; therefore, application of weedicide can be minimized to protect the soil and soil biota including microorganisms, invertebrate and other small animals, thus the soil microfauna diversity thrived. Implementation of armor is important to protect habitat on the soil and resist the organic matter losses.
Diversity
Soil biodiversity reflects diverse range of living organisms ranging from invisible microorganisms, meso-fauna and macro fauna within a soil. Plant roots could also be considered as soil organisms because of their symbiotic relations and interactions with other soil components. Soil organisms play important roles in nutrient cycling, regulate the dynamics of soil organic matter, soil carbon sequestration and greenhouse gas emission, modifying soil’s physical structure and water regimes, improve the nutrient use efficiency and enhance plant health (FAO, 2022). Increasing soil biodiversity contributed to soil resilience enhancement against environmental challenges.
Soil health can be promoted by increasing the soil biodiversity. Application of organic input, reduction of inorganic input application, and reduction of tillage act together to promote biological activity which resulted in increased resilience in extreme events. Cultivation of multi-crops instead of mono-crop could enhance the soil biodiversity and improve soil health. Cultivation of cover-crop and mulching also contributed to soil biodiversity. Cultivation of host crop, such as Prosopis juliflora L., assisted the nutrients absorption for sandalwood (Santalum album L.). The sandalwood-Prosopis combination has been found to improve the vegetative growth and physiological functions of sandalwood (Srikantaprasad et al, 2022). Combination of all the factors mentioned above can improve soil biodiversity and enhance plant health that contribute to soil resilience and increase productivity (SRDP Farm Advisory Service, 2019).
Living roots
Roots play important roles not only for the plant’s physical anchoring on the soil, but also contribute to soil health. Roots provide structure for soil to stick on during adverse conditions such as heavy winds or torrential rains, thus risk of erosion and flash floods can be averted. Roots also help in removing soil particles from its surroundings and made soil less compacted and more porous. Roots also percolate water until the underground water source. Roots also behave as intermediate for nutrient exchange from soil to plant and carbon (carbohydrate) from plant to soil. Roots excreted 10-40% of the photosynthate in the form of exudates that mainly contains carbohydrates, amino acids, organic acids, proteins, sugar, phenolic compounds and other substances. The exudates have many functions such as solubilizing nutrients such as phosphate or potassium from soil particles, change the redox state of the root surface chelate iron and zinc, and desorb nutrients from clay surfaces. Microorganisms such as bacteria and fungus feed on the sugars excreted by exudates thus can thrive in the soil and contribute to soil health. Soil microorganisms such as rhizobia and arbuscular mycorrhizal fixes atmospheric nitrogen for plant consumption. Thriving of indigenous beneficial microorganisms such as biofertilizers and biopesticides strains could offset the negative effects of pathogens through assisting plants in defence against pathogens and diseases. Optimal population of indigenous biopesticide strains make the soil suppressive against pathogens, thus lessen the usage of chemical pesticides that indirectly contributed to soil health. Plant roots complete a cycle in the soil. The deeper the root penetrates; they provide more benefits to soil fertility and carbon storage in the soil. When alive, roots redistribute carbon and nutrients throughout the soil profile. When roots die, it decays and leaves more organic matter which contribute to more stable and longer lasting soil organic carbon.
Plant-animal integration
Plant-animal integration system is an approach where livestock was raised together with crops in farms. Various integrated systems such as sod-based farming, cover crop as forage, ley farming, pasture cropping, dual purpose cereal crops and agroforestry and alley cropping are suitable for integrated crops-animal farming (Lal, 2020). The residue generated from the crop production can be used as fodder for animals, while the manure and animal urine generated can be applied as organic fertilizer for crop production. The animal manure could improve the organic matters level in the soil. This plant-animal integration is efficient in carbon sequestration and reduces the enteric gas emission.
Crop cultural practices
Various environmentally friendly crop production practices in place as improvement of the original green revolution practices. Conservation agriculture, biological agriculture, natural sequencing farming, integrated nutrient management (INM), site-specific nutrient management (SSNM) and circular farming among the agricultural practices that reduces the bad impact of continuous implementation of conventional farming.
Conservation farming
Conservation farming is an approach in which soil management practices are reduced to a minimum, thus preserving the soil properties and nature’s biodiversity. Implementation of this practice contributed to the conservation of soil, water and soil moisture. It enhances fertilizer and seed uses which saves time and money. Main principles of this approach are minimum soil disturbance, crop residue management (leaving previous crop residue in the field or planting cover crops) and crop rotation practices. The soil is tilled just enough to be possible to sow seeds and leave at least 30% of soil surface covered by crop residue or cover crop (Agrivi, 2022).
Biological farming
Biological farming is a system that works closely with nature which balances the soil to produce healthy, pests and disease resistant crops while reducing the use of chemicals. The basic principles of the biological farm are balanced application of nutrients, fertilizers that possess less damage to soil life and plant roots, responsible application of pesticides and herbicides, create maximum plant diversity by using green manure and crop rotation, maximize decay of organic matters and supply the soil with carbon from compost, green manures, livestock manures and crop residues. This practical system offers a sustainable and profitable agriculture practice that also promotes the soil health (Midwestern BIOaG, 2022).
Circular agriculture
Circular agriculture focuses on the use of minimal amounts of external inputs, closing nutrient loops, regenerating soil and minimizing the impact on the environment. Circular agriculture aims to address the rising concerns about the unsustainability of global food production, soil destruction, loss of biodiversity and depletion, degradation and pollution of water and land resources. Circular agriculture supports a food system from growing to harvesting, packing, processing, transporting, marketing, consuming and disposing of food that are designed with a view of sustainable development (United Nations, 2021). Circular agriculture encourages mixed crop-livestock and organic farming, agroforestry and water recycling and wastewater reuse which are aimed to reduce CO2 emissions, more efficient utilization of natural resources and minimize the use of input significantly. As an example, circular agriculture can be employed by combining crop cultivation with animal husbandry where the wastes generated from the crop cultivation either can be used as fodder for animal or composted as organic fertilizer. The manure and urine generated from animal husbandry can be used as organic fertilizer or can be used as amendments for crop residues composting. If the farm has fish ponds, the water from the pond can be used to irrigate the crops, in return the crop residues can be used as fodder for the fish. Agroforestry is another strategy which combines tree planting with crops or pastures. The pasture can be used for farm animal grazing. Recycling and reuse of irrigation is an important part of circular agriculture water management which addresses the water scarcity issues.
Circular agriculture is more sustainable with less inputs as opposed to the big scale monoculture farming. However, this circular agriculture is labor intensive, therefore it is not suitable for big-scale farms. This system is suitable for smaller scale farms mainly for food production which contributes to food security in the rural areas and poor countries. This system promises resilient agriculture that contributes to soil health improvement.
Organic farming
Organic farming is an agricultural system which uses ecological approach in pests and diseases control and biologically derived fertilizers mainly from animal and plant wastes and nitrogen fixing cover crops. Modern organic farming does not use chemical pesticides, reduces soil erosion, decreases nitrate leaching into groundwater and surface water, and recycles back animal wastes into farms. The soil organic matter level will increase and the soil will become healthier with continuous organic farming. Circular agriculture is best suited for organic farming provided that organic inputs and pest and disease control measures are applied.
Integrated Nutrient Management (INM)
Integrated Nutrient Management (INM) is an approach that manages both organic and inorganic plant nutrients for optimal production of cultivated crops, forage and tree species, while conserving the natural resource base that is essential for long term sustainability (Smalling, 1993). Organic nutrient inputs can be obtained from plant residues such as rice straw or Plant Growth Promoting Rhizobacteria (PGPR). Compost generated from crop residues is a nutrient rich organic fertilizer that can be easily produced at the farm level at a very low cost. PGPR is defined as a heterogeneous group of non-pathogenic free-living bacteria that are associated with plant roots and mediate improvements in plant growth and/or health (Kloepper et al., 1989; Bent, 2006). The PGPR contributes by providing nutrients for plant growth including atmospheric N2 fixation, releasing of P from soil and organic matters, chelates ferric ion from soil, improves the plant root system, and reduces biotic and abiotic stress which can decrease the plant’s vigor and health. The combined usage of organic fertilizers, decreased amounts of chemical inputs, and the addition of biofertilizers could significantly improve nutrient management in crop production and reduces the dependence on imported chemical fertilizers. Based on our own experience, the application of 50% of recommended rate of chemical fertilizers and biofertilizer combination managed to produce yield as good as 100% of the recommended rate of chemical fertilizer alone. Based on 3 years of extensive participatory observation in the Mekong Delta showed reduction in fertilizer needs as high as 52% in rice production (Nguyen et al, 2017). This would in turn reduce the spending in the purchase of chemical fertilizers thus saving on production cost. In addition, this INM approach can also promote an environmentally friendly and sustainable agriculture practice by reducing environmental contamination. As an added benefit, the usage of PGPR also helps the environment by reducing greenhouse gas emissions from agriculture.
Site Specific Nutrient Management (SSNM)
Fertilizer application recommendation are often determined based on crop response data averaged over large area although farmer’s fields show large variability in terms of nutrient supplying capacity and crops response to nutrients (Richard et al, 2022). Thus, blanket application of fertilizer may lead to over-fertilization in some areas and under-fertilization in others, or the application of an improper balance of nutrients for the soil or crops. As an alternative for blanket fertilizer application, Site Specific Nutrient Management (SSNM) aims to optimize the supply of soil nutrients over time and space to match the requirement of crops through four principles namely right product, right rate, right time and right place (Richard et al, 2022). The selected fertilizer products such as commercial fertilizers, livestock manure, compost and crop residue must ensure balanced supply of nutrients and suits the soil properties. The rate of fertilizer application is determined by the fertility status of sites determined through soil test. The optimal time for fertilizer application is determined based on nutrient requirement for different growth stages. The application place for fertilizer such as placing and keeping nutrients at optimal distance from the crop and soil depth could minimize the nutrient losses. The amount of nutrients will be varied based on the nutrient availability in the soil. Based on Witt and Dawe (2002) experience, the productivity of rice in SSNM plot can be continuously increased to 4 seasons with efficient nutrient application. In Malaysia, implementation of SSNM with optimum nutrient application determined by using RiceFert modelling system had increased the rice production (DOA, 2019).
Rehabilitation of arid soil that is unfit for agriculture
The size of desert in Asia amounted 3.83 million square km which covers the Arab Peninsula, Gulf countries, Iran, Iraq, China, India, Mongolia and Pakistan. These arid soil structures are very poor and receive very little rain making it unsuitable for crop production. However, latest invention by using clay and glue had improved the sand structure that makes it suitable for crop production. United Arab Emirates, Saudi Arabia and China were among the countries which had adopted this technology to rehabilitate their desert and crop production. In Malaysia, tin mining had produced sand tailing which is totally unfit for crop production. However, this unfit soil can be rehabilitated by burying empty fruit bunch or compost, which made it fit for cultivation of vegetables, tubers and small size fruit trees. Rehabilitation of deserts and sand tailings offer more land area for crop production which will generate extra food and contribute to food security. These rehabilitation technologies bring a new ray of hope for food security.
CONCLUSION
Intensive conventional agriculture practices had caused many adverse effects that directly deteriorate the soil health, decrease soil fertility and created environmental issues. Restoration of this degraded soil is urgently needed for crop production and fulfil food demand of an increasing population. Various, strategies are available and proven working for the restoration of degraded soil. It is still not too late to rehabilitate this unhealthy soil and turn it as productive crop farms. Failure to react now in order to restore the degraded soil will push to an irreversible state of degraded soil. Rehabilitation of soil that is totally unfit for crop production such as desert and sand tailing shed a new ray of hope for global food security and humanity.
REFERENCES
Agrivi. 2022. How can conservation farming reduce climate change? Preprint at https://www.agrivi.com/blog/how-can-conservation-farming-reduce-climate-change/ 2022/07/25.
Blanco-Canqui, H. and Lal, R. 2010. Soil resilience and conservation. P. 425-447. In Principles of Soil Conservation and Management, 425-447, Springer, Dordrecht. https://doi.org/10.1007/978-1-4020-8709-7_16
Doran, J.W.,and Zeiss, M.R..2000. Soil health and sustainability: Managing the biotic component of soil quality. Applied Soil and Ecology, 15, 3-11.
DOA. 2019. Pengurusan Nutrient Lokasi Spesifik: Jelapang Padi Pulau Pinang.pp 172, DOA Printing, Putrajaya.
Earth Worm Foundation. 2022. Look Down Earth. Preprint at https://www.earthworm.org/campaigns/lookdowntoo?gclid=CjwKCAjwwo-WBhAMEiwAV4dybdT2f_3If_ZVId6fxulGlaIYQ39faJ_1Jb6PFDYi8po-yWZRFYcuMRoC-WEQAvD_BwE/ 2022/06/30
FAO. 2015. Status of World’s Soil Resources – Main Report. Chapter 10: Regional Assessment of Soil Change in Asia. FAO, Rome, Italy, pp. 329.
FAO. 2022. FAO Soil Portal. Preprint at https://www.fao.org/soils-portal/soil-biodiversity/en/ 2022/07/14
Guo, M. 2021. Soil health assessment and management: Recent development in science and practices. Soil System 5, 61.
Kloepper, J.W., Lifshitz, R. and Zablotowicz, R.M. 1989. Free-living bacterial inocula for enhancing crop productivity. Trends in Biotechnology 7, 39-44.
Lal, R. 2020. Integrating animal husbandry with crops and trees. Frontiers in Sustainable Food Systems 4:113 doi: 10.3389/fsufs.2020.00113
Midwestern BIOaG. 2022. About Biological Farming. Preprint at https://www.midwesternbioag.com/about/ about-biological-farming/ 2022/07/25.
Moebius-Clune, B.N., Moebius-Clune, D.J., Gugino, B.K., Idowu, O.J., Schindelbeck, R.R., Ristow, A.J., van ES< H.M., Thies, J.E., Shayler, H.A., McBride, M.B., Kurtz, K.S.M., Wolfe, D.W. and Abawi, G.S. 2016. Comprehensive Assessment of Soil Health – The Cornell Framework, Edition 3.2, Cornell University, Geneva, NY, pp.134.
Nguyen, T.H., Phan, T.C. Choudhury, A.T.M.A, Tose, M.T, Deaker, R.J. and Kennedy, I.R. 2017. BioGro: A Plant Growth Promoting biofertilizer validated by 15 years’research from laboratory selection to rice farmer’s fields of the Mekong Delta. In Agro-Environmental Sustainability, Ed. J.S. Singh and G. Seneviratne (eds), 237-254. DOI 10.1007/978-3-319-49724-2_11
Richards, M.B., Buttetbach-Bahl, K., Jat, M.L., Lipinski, B., Ortiz-Monaterio, I. and Sapkota, T. 2022. Site-Specific Nutrient Management: Implementation guidance for policymaker and investors. Global Alliance for Climate-Smart Agriculture (GASCA), pp.10. Preprint at https://ccafs.cgiar.org/resources/publications/site-specific-nutrient-management-implementation-guidance-policymakers/ 2022/07/26
Setboonsarng, S. & Gregorio, E. 2017. Lage scale soil health restoration: The way forward for reversing climate change while enhancing food and nutrition security. ADB Southeast Asia Working Paper Series, No. 16, pp. 31.
Seybold, C.A., Herrick, J.E. and Bredja, J.J 1999. Soil resilience: A fundamental component of soil quality. Soil Science, 164(4): 224-234.
Smaling, E.M.A. 1993. Soil nutrient in Sub-Saharan Africa. In: The Role of Plant Nutrients for Sustainable Food Crop Production in Sub-Saharan Africa, eds. H. van Reuler and W.H. Prins, pp. 53-67, Leidschendam, the Netherlands: Vereniging van Kunstmest Producenten.
SRDP Farm Advisory Service. 2019. Technical Note 271: Soil biodiversity and soil health.pp. 5. Preprint at https://www.fas.scot/downloads/tn721-soil-biodiversity-and-soil-health/ 2022/07/03
Srikantaprasad, D., Malliarjuna Gowda, A.P., Pushpa, T.N., Thimmegowda, M.N., Umesha, K., Ravikumar, R.I and Prasanna, K.T. 2022. Identification of suitable host for sandalwood cultivation in Northern dry zone of Karnataka. Industrial Crops and Products 182: 114874 (in press)
Srinivasarao, C., Kundu, S., Subha Lakshmi, C., Sudha Rani, Y., Nataraj, K.C., Gangaiah, B., Jaya Laxmi, M., Vijay Sankar Babu, M., Rani, U., Nagalakshmi, S. and Manasa, R. 2019. Soil health issues for sustainability of South Asia Agriculture. EC Agriculture 5.6.
Taufik, A. M., Zaman, O. M. F., Fauzi, M. I. M., Khusairy, K.M., Herman, S.E., Hashim, A.M., Azlan, O., Saifulizan, M.N., Bunyamin, A.K. and Mansor, N. 2021 Effect of mole drain and tracked agricultural prime movers on soft soil paddy areas. Advances in Agricultural and Food Research Journal, 2(2): a0000228. Preprint at https://doi.org/10.36877/aafrj.a000022/2022/07/03.
Triplett Jr., G.B. & Dick, W.A. 2008. No-tillage crop production: Revolution in agriculture. Agronomy Journal 100: S1153 – S-165. Preprint at https://doi.org/10.2134/agronj2007.0005c/ 2022/06/30
Tumma, M.K and Poorna Chandrika, K.S.V. 2022. Zero tillage Zero worries. Vikaspedia. Preprint at https://vikaspedia.in/agriculture/best-practices/sustainable-agriculture/crop-management/201czero-tillage201d-zero-worries/ 2022/07/22
USDA-NRCS (2019). Soil Health; Natural Resource Conservation Service, U.S. Department of Agriculture: Washington DC, USA, 2019. Preprint at https://www.nrcs.usda.gov/wps/portal/nrcs/detail/pa/soils/health/?cid=nrcseprd1200408/ 2022/07/18
United Nation (2021) UN/DESA Policy Brief # 105: Circular agriculture for sustainable rural development. Preprint at https://www.un.org/development/desa/dpad/publication/un-desa-policy-brief-105-circular-agriculture-for-sustainable-rural-development/ 2022/07/22
Witt, C. and Dawe, D. 2014. Performance of Site-Specific Nutrient Management in intensive rice cropping systems of Asia. Better Crops International 16 (1): 25-30.