ABSTRACT
The population of the world is ever-growing. It is expected to reach 10 billion in 2050. To feed all those people, significant improvements in our food production systems are required. Current agricultural practices have proven damaging to ecological systems worldwide, leading to greenhouse gas emissions, deforestation, loss of biodiversity and water pollution, among other problems. Moreover, two-thirds of agricultural land is currently used to feed livestock. We have already overstepped the Planetary Boundary as defined by Rockström et al for reactive nitrogen usage. Simply upscaling agricultural activities to meet the demands of the growing population is therefore not an option. Such an approach would lead to a Nitrogen fertilizer requirement which is three times higher than the world can sustain. We therefore need to become three times more efficient in using Nitrogen and consciously evaluate the way we produce food. New and disruptive innovations are needed to ensure a sustainable future for the next generations. This paper describes small-scale grass biorefinery technology which will contribute to solving three major issues: agricultural nitrogen emissions, the need for more agricultural land and the import of soy. Grass can be fully upgraded to the following components: (1) Refined grass: highly digestible grass which is high in fiber and rumen undegradable proteins, which is ideal as roughage for ruminants (dairy cattle, cattle, rabbits) or horses; (2) Grass protein concentrate: dried protein concentrate that is readily digestible with an optimal amino acid composition and which is an appropriate alternative to environmentally harmful soy meal in chicken, piglet, petfood or fish feeds; (3) FOS: Fructo Oligo Saccharides, a soluble fiber that acts as a prebiotic which improves digestion, stimulates the immune system, generally improves animal health and wellbeing and lowers the need for antibiotics; and (4) Mineral concentrate: which is rich in potassium and other minerals and in organic nitrogen which can serve as an organic, soluble, plant-based alternative for chemical or animal-derived fertilisers. The technology can be economically operated in grass rich countries at a scale of 8 MT of fresh grass per hour. In developing countries, it can be operated economically on a scale of 0.4 MT per hour. Both systems can also process green leaves of other plants which are not currently used to produce food or feeds: leaves of cassava, palm leaves, sugar beet leaves, green leaves of rice collected just after the rice harvest, etc. When grass derived from marginal land is included, we can increase the production of protein without increasing the land required for agricultural cropping. Since the grass biorefinery splits soluble and insoluble proteins, the first can be fed to cattle and the latter to pigs and poultry because in so doing the efficiency by which these proteins are used increases by 50%. This again means that one-third less land, and one-third less N, P, K fertilizers are required. As 50% more animal protein can be produced from the same hectare of grassland more economical value will be created which can be divided over the players in the chain: crop and grass farmers, biorefinery and animal farmers.
Keywords: Nitrogen Planetary Boundary, Biorefinery, Grass, Small scale, Protein
INTRODUCTION
The population of the world is ever-growing. It is expected to reach 10 billion in 2050. To feed all those people, significant improvements in our food production systems are required. Current agricultural practices, however, have proven damaging to ecological systems worldwide leading to greenhouse gas emissions, deforestation, loss of biodiversity and water pollution, among other things. Moreover, two-thirds of agricultural land is currently used for feeding livestock. Simply upscaling agricultural activities to meet the demands of the growing population is therefore not an option. We need to become more efficient and consciously evaluate the way we produce food. New and disruptive innovations are needed to ensure a sustainable future for the next generation.
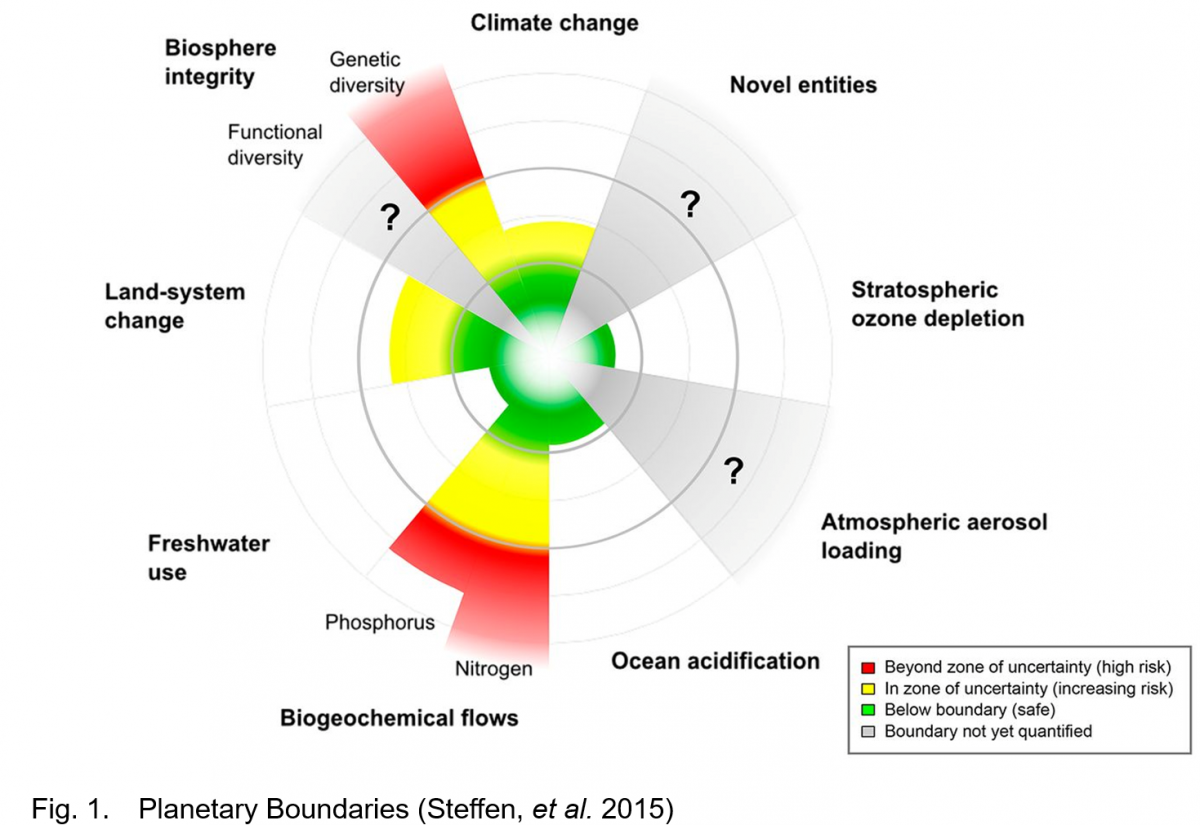
The ecological capacity of our planet is coming under increasing pressure. The emission of greenhouse gases obviously contributes to global warming, however that is not the only problem, unfortunately. Johan Rockström and his team defined several ‘Planetary Boundaries’ in a 2009 model, which was updated in 2015 (See Fig. 1). This model visualises the severity of different threats to the planet. This research indicates that the level of nitrogen emissions may present an even bigger problem to our planet than the emission of greenhouse gases. This problem is largely rooted in agriculture and our unsustainable food and feed production systems. The use of nitrogen as a fertilizer and for chemical products has brought us enormous benefits in the past century. However, losses of fertilizer nitrogen and NOx from combustion processes to the environment lead to many side effects on human health, ecosystem health and the climate.
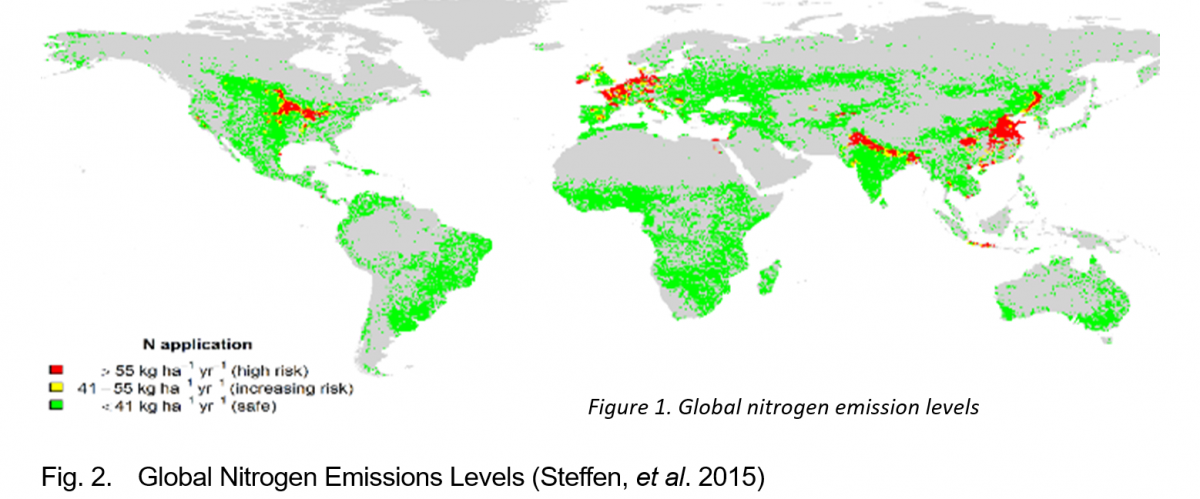
Agriculture is an important source of reactive nitrogen in the atmosphere and water bodies. Currently, humans produce 131 million MT of ammonia globally each year, which is about 50 million MT more than the planet can sustain. Around 75% of these emissions are estimated to originate from livestock farming. The consequences include soil acidification, loss of biodiversity and ‘algae blooms,’ resulting in anoxic zones in the oceans. Figure 2 provides a global representation of the areas with greatest excess nitrogen use per hectare. In the Netherlands, for example, the nitrogen crisis has already become a cause of major concerns in the past year, with at least 35% of all nitrogen losses to nature stemming from agricultural practices. One of the proposed solutions to this problem is to decrease the amount of livestock held by farmers, and although livestock is accountable for the majority of ammonia production through manure and urine wastes, half of the total Dutch cattle herd would need to be halved in order to reach acceptable emissions of nitrogen. This would, however, have serious consequences for thousands of farmers who rely on livestock herding for their livelihood and is also damaging to the Dutch economy. The same could be argued for many other countries whose economies depend partly on livestock farming and export of agricultural produce.
In Ireland, for example, there has been a mandatory ammonia emission target for agricultural businesses since 2010. From 2030 onwards, ammonia emissions must be reduced to 1% below 2005 levels by 2020 and 5% below 2005 levels. Agriculture is responsible for most of these emissions, and in recent years, Ireland’s ammonia emissions from agriculture have been increasing (Department of Agriculture, Food and the Marine). The Irish government has already proposed several methods for reducing ammonia emissions, among which include adapting livestock feeding strategies by extended grazing, maintaining the quality of crude protein when making silage or use of low-crude protein in animal feeds.
In France, studies on nitrogen emissions have been performed as well. As a result, livestock production has been identified as a major contributor to national ammonia, methane and nitrous oxide emissions. Farmers have been advised to take these emissions into account as part of efforts to mitigate climate change and improve air and water quality, when considering changes in farming practices such as feeds, types of livestock buildings, emission treatment, etc.(Hassouna and Eglin).
By using some 130 Mmt of nitrogen fertilizer in agriculture, worldwide, we have already overstepped the Planetary Boundary. With a population growing to 10 billion by 2050 which means we will eat more meat, this problem will become even much larger. Taking the efficiency of European protein-production for human food which requires 7 kg N input to obtain 1 kg N in food-protein to feed people with the required minimum of 50 grams per day, we will need an amount of Nitrogen fertilizer equivalent to 3 times the Planetary Boundary in 2050 (Table1). These numbers will be even larger when we realize that many western people consume 100 grams of protein or more. To stay within Planetary Boundaries the Nitrogen Use Efficiency (NUE) needs to be raised from 15% to over 35%, and the intake of protein should be reduced to 50 gram/person/day. While currently two-thirds of good agricultural land (1500Mha globally) is used for animal feed production, much of this feed should be produced on marginal lands to limit use of good agricultural land to direct human food production and in order to reduce further loss of biodiversity, another Planetary boundary under pressure.
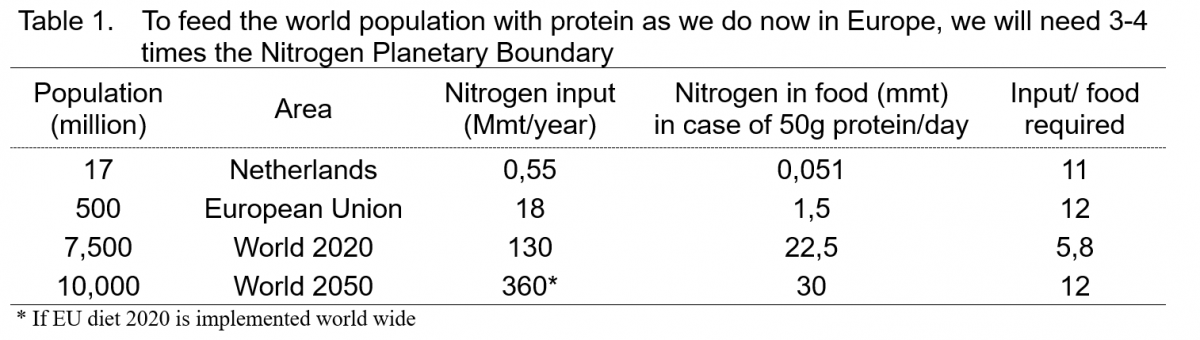
SOLUTIONS TO STAY WITHIN PLANETARY BOUNDARIES
Halving the livestock herd in order to reduce nitrogen and ammonia emission levels would undoubtedly be a drastic measure, which might not be the most desirable way to go when considering the ever growing demand in, for example, meat and dairy products for food-grade proteins for a growing and more prosperous human population. Other measures such as more efficient feeding and development of alternative protein sources could prove to be an effective solution, without increasing demand for agricultural land or damage to rural economies.
This can be achieved by matching the amount and quality of nutrients consumed to that needed to meet the animals’ requirements and increasing the efficiency of utilization of the nutrients consumed. Currently, rations are often formulated with a ‘safety margin’ and based on mixing existing raw material streams within economic limits, which means the concentrations of nutrients are increased beyond the actual needs, sometimes by as much as 30 to 50%. This results in the production of excess nitrogen, phosphate and other residuals. Although composition can be good, the digestibility might be poor, which again leads to an oversupply of components in animal feeds. Reducing the excessive levels of nutrients and improving the efficiency of nutrient utilization, could therefore prove to be a simple, yet effective solution for the impending Global nitrogen crisis.
Matching the applied amount and quality of feeds can be achieved by adding essential amino acids to monogastric feeds to reduce the total amount of protein in the diet. Even more powerful is the splitting or refining of different components present in the actual feed raw materials, in order that proteins, fats, carbohydrates, minerals, vitamins, water and other required components are provided in a tailormade way to the animal throughout its life. This biorefinery approach exemplified in this paper with a focusing on grass and other leafy materials can also be applied more widely for most raw materials now utilized as animal feeds. Obviously, there are costs and energy requirements associated with this approach, and these will be addressed in this paper as well. Aside from reducing Nitrogen and phosphate emissions, less agricultural land is required when the biorefinery approach is utilized. Other benefits such as improved biodiversity and a reduction in transport of soy and raw materials are observed. The amount and volume of manure with concomitant emissions of NH3 and methane will also be reduced.
To cope with the Planetary limitations holistically, land use should also be optimized with yield gaps closed, while land that is suitable for crops for human consumption used for that purpose, with marginal lands such as wetlands, used for animal feed production. In the event that fertilizers are used, these should be applied in a way that limits losses to the environment or to the deeper layers in the soil. Legumes, the group of plants that can fixate nitrogen from the air using bacterial nodules on their roots, generally have much lower losses of reactive nitrogen because this is produced close to the roots. Vegetable crops in the Netherlands often have a fertilization efficiency of less than 30% which can be improved by drop irrigation. Once proteins are formed, one should realize that their value is much higher than when these proteins would be used as nitrogen fertilizer, by leaving agricultural residues on the field containing this protein. The same reasoning holds for animal waste streams which may face more difficult reuse limitations for safety and hygiene reasons. It is inevitable that animal manure contains minerals. These can be recycled to agriculture if there is no excess of these minerals. In cases where mineral excesses exist, such as in the Netherlands, ammonia can be stripped from anaerobic digested manure with technology that has been developed e.g. by a Dutch company Byosis, an approach that is now in operation in various countries around the world.
Finally, we can adjust the need for reactive nitrogen which limits the amount of daily protein consumed to the minimal requirements of about 50 grams, while in many western countries, the consumption of protein is more than double per head of population.
In 2017, the EU used an estimated 34.4 million MT of soybeans, soymeal and soybean oil, approximately 12% of global soybean production (European Soy monitor). By far the biggest proportion of this soy (30.8 million) was used as soymeal for agricultural purposes. As soy grows in temperate, subtropical and tropical climates, its use is mostly dependent on imports. Most of its production is concentrated in North and South America and aside from China, the EU is its biggest customer. Currently, around 85% of the global soybean harvest is crushed, resulting in around 78.5% soymeal and 18.5% soybean oil. The oil is used for varying purposes, while virtually all soymeal is used in compound feeds. Soy’s global success can be explained by the fact that it is a low cost, storable and transportable protein, as a side product of soy oil. Soy has, consequently, been one of the most important drivers of regional economic growth in Argentina, Brazil and Paraguay. As a result, the soybean industry is also connected to a range of environmental and social sustainability issues in the producing countries. Rapidly growing amounts of landmass have been dedicated to soy cultivation in the past few decades, leading to deforestation (more than 792,000 square kilometres of rainforest have been lost since 1970 in Brazil alone) and conversion of natural ecosystems.
Six factors are essential to optimize NUE and landuse at the same time:
- fertilization efficiency of the crop, including redistribution of N;
- protein yield per ha.year;
- digestability and feed conversion to animal protein, which includes redistribution of proteins over various different animals;
- digestability and availability of essential amino acid in human food (PDCAAS);
- how much protein is consumed per person perday; and
- land (un)suitable for food crops.
While in European husbandry the nitrogen inputs are seven times higher than the nitrogen that ends up as protein in animals and vegetative consumer products, the reduction of losses will improve the efficiency of the animal feed system (Leip, et al 2013) According to Lesschen and Sanders, the Nitrogen use efficiency can be improved by a factor two in the Netherlands by the year 2050 (Lesschen and Sanders). This will be accomplished by reducing ammonia emission from manure storage and stables, and growing catch crops in the same season directly after corn. Protein will be extracted from these catch crops by biorefinery technology. This biorefinery technology will be applied to grass as well. Biorefining the raw materials that nowadays are used as animal feeds, will not only improve the N use efficiency, but will also enable the use of feed components such as protein at the highest value, e.g. in pig or poultry while cattle will benefit less from such protein. Additional components with no value for animals can be used to substitute fossil resources or to improve fertilizer use efficiency. This will reduce overall greenhouse gas emissions.
In this paper we will introduce the biorefinery- process and the products that can be obtained from grass and from several other sources. Furthermore, we will report several animal feeding results obtained with the products from the biorefinery.
GRASS BIOREFINERY
The Dutch company, Grassa has developed a bio-refinery process that upgrades grass and vegetable green residue streams to new and high-quality components that can serve as compound feed ingredients. Although the refinery process is suited for processing various raw materials (e.g. grass, beet leaves or pea plants), Grassa will initially focus on grass, clover and vegetable wastes. For simplicity, we will simply refer to those raw materials as ‘grass’ throughout this application.
Grassa biorefinery products
Through biorefining, grass can be fully split into the following components:
- Refined grass: highly digestible grass high in fiber and rumen resistant proteins, which is ideal as roughage for ruminants (dairy cattle, cattle, rabbits) or horses. As compared to silage, it has a constant dry matter of 35% and it has a higher occurrence of resistant protein than is the case in grass silage. This is beneficial for improving the nitrogen use efficiency since non-resistant protein is broken down in the rumen and subsequently converted to microbial protein of which a considerable part is not digestible by the cow as is also the case for the microbial nucleic acids. The cow needs microbial production for a better digestion, but not too much.
- Grass protein concentrate: dried protein concentrate that is readily digestible, has an optimal amino acid composition, is non-GMO, rich in carotenoids and omega-3 fatty acids and low in minerals and is an appropriate alternative for environmentally harmful soy meal in poultry, pig, petfood or fish feeds. Eventually it could even be processed to human food, as a partial replacement of meat, dairy or egg protein in our diets. Currently, compound feed production relies heavily on the import of soy as a source of protein. Ignoring its considerable and damaging ecological side effects – i.e. deforestation, erosion, water pollution, loss of biodiversity, CO2-emissions, high pesticide and herbicide usage and global transportation – soy has a a good combination of essential amino acid composition and high digestibility ( 80%). In particular, soymeal is a low-cost raw material that has been widely accepted as a source for protein in animal feeds, as it consists of 45-48% protein of dry matter. A common higher quality alternative for soymeal is soy protein concentrate, which contains about 60-70% protein, and soy protein isolate as high as 90% protein These products are of higher quality, but are also a lot more expensive: soymeal is sold for 440 US$ a MT, soy isolate for about 1000US$ a MT. Moreover, it has the same impact on the planet. As a result of the high costs and associated environmental impacts, animal feed producers are now searching for local alternative protein sources. Bio-refined grass would make an excellent replacement for soy, as it is similar in protein content (ca. 45-55%) and has an even higher protein digestibility (94%) but lacks all ecological downsides.
- FOS: Fructo Oligo Saccharides, a soluble fiber that acts as a prebiotic that improves digestion, stimulates the immune system, generally improves monogastric animal health and wellbeing and lowers the need for antibiotics.
- Mineral concentrate: which is rich in potassium and other minerals and in organic nitrogen and can be added to compound feed or serve as an organic, soluble, plant-based alternative for chemical or animal-derived fertilizers. This concentrate consists mostly of potassium and nitrogen. The nitrogen is mostly present in the form of amino acids, which is beneficial for plants because with it they will need less energy to build in nitrogen.
With these products, Grassa’s serves as an economically viable solution with a sustainable business case which also enhances food security for humans and animals. Through the refinery process we enable the local production of proteins, which is good for rural development, in a sustainable and circular way and without any use of pesticides. Grassa’s products are a great source of locally produced and easily digestible proteins and fibers, which are in high demand in the strongly growing organic agricultural sector. In some places, the demand for proteins and fibers is so high that it can barely be met. Organic/biological animal farming in Germany, for example, requires the use of exclusively German-produced animal feed raw materials. Bio-refining of biological grass makes it possible to meet the ever-growing demands for fiber and protein.
In addition, bio-refinery provides an elegant solution for the reduction of nitrogen emissions in the form of ammonia. In the Netherlands, we can now obtain approximately 50% higher yield of animal proteins per hectare, which now produces 12,000 kgs of milk, through the biorefinery approach which opens the structure of grass and fractionates the proteins present.
Grassa Bio-refinery technologies
Grassa developed various technologies one on 2 MT/hour capacity (Fig. 3) that is mobile, but the larger capacities still can be transported to other locations but several lorries will be required. The process that uses different unit operations (Fig. 4) for refining grass, such as pressing, protein extraction, nano-filtration and reverse osmosis. The grass is crushed and pressed first, separating juice from the grass fibers. The following process comprises several steps. The refined grasspresscake is compressed and sealed airtight for the purpose of conservation. The juice contains part of the nutrients (proteins, carbohydrates and minerals) and is processed by extracting and concentrating the proteins. The fluid that remains is further processed and is separated in a sugar fraction, a mineral rich fraction and clean water. The bio-refinery installation is designed in a small to medium size for local or regional processing of biomass. Although the focus for deployment of this technology is currently on cultivated and natural grass, clover and vegetable waste streams, in the future practically all types of fresh green materials or leaves could be processed using these installations and technologies.
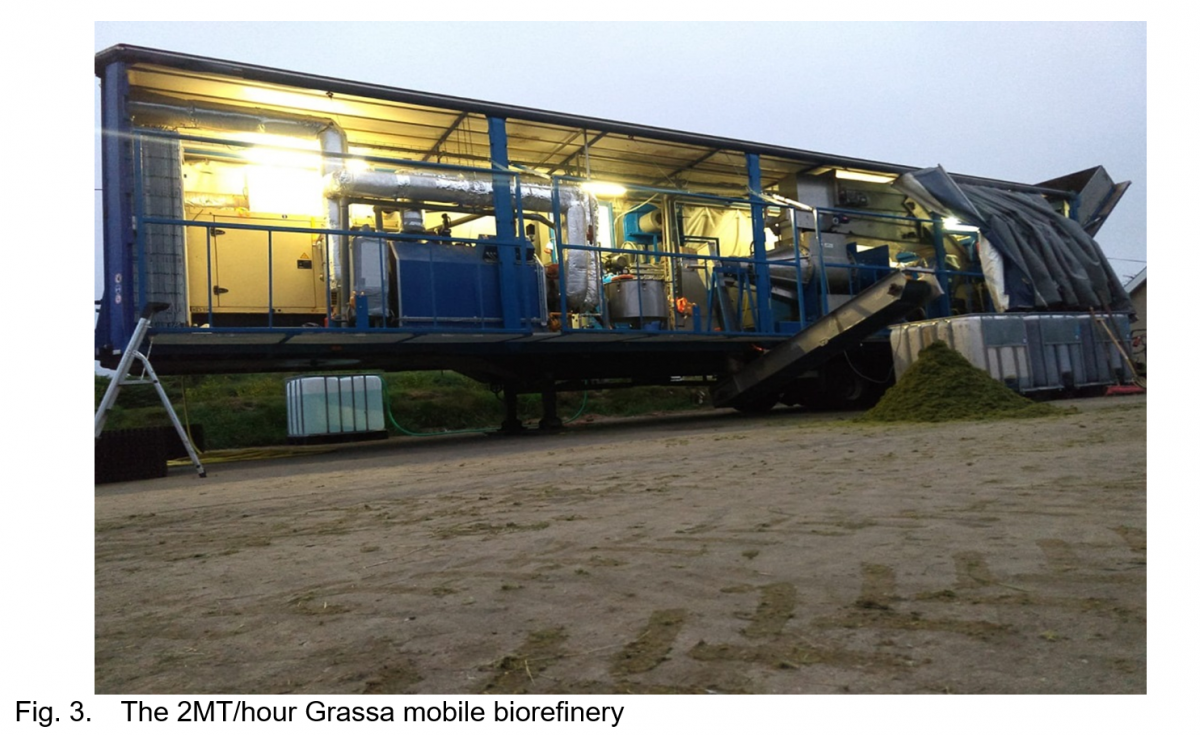
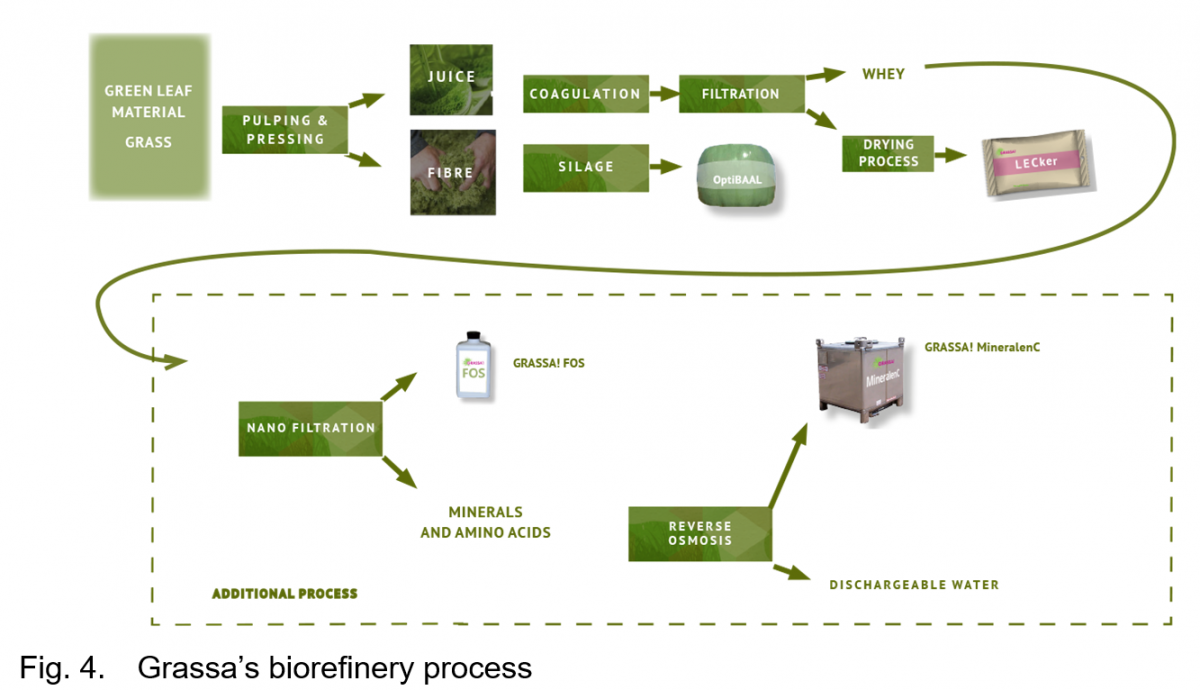
Grassa focuses on ‘green’ bio-refinery which means that they solely use separation technology in their processes. Grassa uses an extruder, coagulation machine and belt filter, protein separation and washing, nano-filtration, reverse osmosis and a protein dryer. As these technologies use no biological or chemical conversions, the products are minimally processed and all the dry matter ends up in one of the four products.
In the Netherlands the processing and operating capacity of the complete process is now 4 MT of fresh leaf material per hour. For 2021 the construction of an 8 MT/ hours biorefinery which can operate under economic conditions in the Netherlands is planned. The complete 8 MT installation can be transported but unlike earlier versions, should not be regarded as movable. The previous 2 MT/hour Demonstration unit which contained only the refined grass and protein concentrate production, is mounted on a trailer and is fully movable. In the summer of 2019 this installation went to Ireland for a six-week demonstration on five commerical farms as part of the Biorefinery Glas project.
For Grassa’s innovation, the concept is that contractors collect grass from farms with grasslands and horticulture with green residue streams whose biomass are traded for refinery in exchange for compensation. A local bio-refinery then converts the grass into four products that Grassa will sell commercially: Refined grass, Grass protein concentrate, FOS concentrate and mineral concentrate. Refined grass will be distributed as an end product to livestock farmers with dairy cows, horses or rabbits, Grass protein concentrate will be supplied in bulk to compound feed companies as a feed ingredient for poultry, pigs, fish and cows, FOS concentrate will be supplied in bulk as a feed ingredient for pigs and the mineral concentrate will be distributed towards greenhouse horticulture as a fertilizer product. In this way, raw material cycles can be closed, and no large transportation movements are needed for nutrients and minerals. The local need for soy imports will also decrease significantly. Moreover, each of the four components that the machines produce, hold considerable benefits in comparison to current products on the market for compound feeds.
Feeding refined grass presscake to cows
The refined grass that has been obtained as press cake in the Grassa process has been fed to cows during trials at Louis Bolk Institute in the Netherlands (Pijlman et al). During this trial control cows were fed with 13 kg silage (dry matter base) and 8.6 kg of concentrates while in the experimental diet, two-thirds of the silage was substituted by refined grass press cake. In Table 2, we see that the Nitrogen input has been reduced from 721 g to 605 g per cow per day but that the nitrogen in the milk is 162 g in both cases. Fecal and urine N excretion have been reduced, therefore. The same picture holds for phosphate.
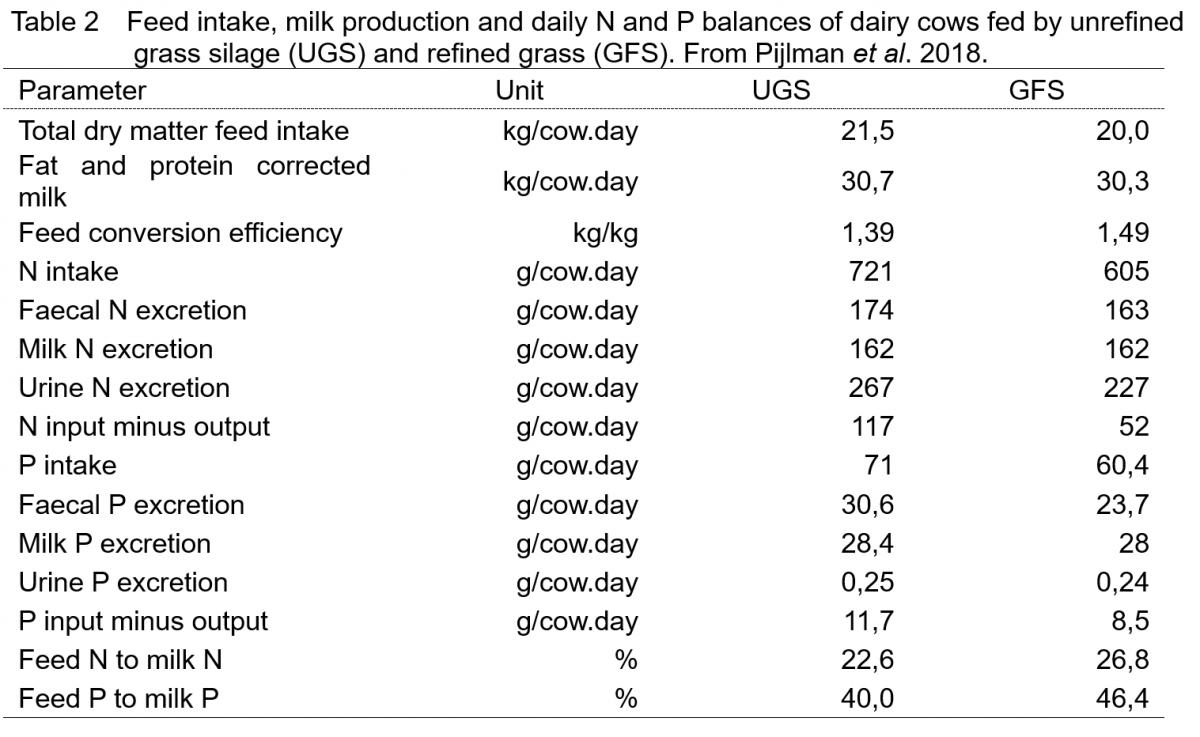
Overall, the feed conversion efficiency increased from 1.39 to 1.49, an increase of 7%. The N and P efficiencies increased by 18% and 12% and if calculated back to the 8 kg of refined grass on the 21.6 kg of the total diet, these numbers would rise to 18%, 45% and 31%, respectively. Part of the explanation of these improvements can be attributed to the ratio of the components in the feeds, which are better adapted to the needs of the cow in the trial sample, whereas in the control sample N and P are given in excess of the cows needs. This can be more fully understood if one realizes that part of the protein fed to cows are degraded and converted to microbial protein. This conversion can be beneficial to the cow if the diet contains protein with low essential amino acids since the conversion to microbial proteins results in a much better essential amino acid composition. However, in the case of grass, the essential amino acid content is as high as in soy protein, then a higher proportion of resistancy is preferred since the microbial resynthesis of protein amounts to as much as a third of nitrogen input losses, due to inefficiencies of rumen microbial protein synthesis, the formation of rumen microbial nucleic acids, and the indigestibility of some of the formed microbial proteins. (Dijkstra, et al. 2013).
In addition, basic biochemistry teaches that for each peptide bond to be synthesized 7 ATP is required. This would require 0.5 kg of sugar for the peptide bonds in 1 kg of protein only, to be resynthesized from 1 kg of plant protein that is degraded in the rumen. Also the other components of the microorganisms also require energy to be (re)synthesized. This additional step in the food chain requires resources and should be considered in a way that is healthy for the animal and optimized for resource use efficiencies.
In the Netherlands, about 75% of grass is ensiled. Another advantage of the grass biorefinery as compared to traditional ensiling is that there are no losses during the ensiling process. In traditional ensiling, the farmer must first wait for two consecutive sunny days in order to dry the grass. Grass biorefinery can be done even during wet weather, so the farmer can better plan his activities and cut the grass at the time the quality of the grass is best. Unlike traditional ensiling, there are no losses in nitrogen during spreading and re-spreading of grass during drying and no losses from leaking grass juices or mall grass particles during the collection after drying. In good farming practices, losses of 15% are indicated but these can well be as high as 25% in some cases. The Nitrogen that is in the protein of this part of the grass will become available as fertilizer after that grass leaf has been degraded in the soil, but of this recycled nitrogen about 40% will be lost since the fertilization yield in grassland is about 60%. In the biorefinery process, the wet grass is immediately processed into grass press cake of approximately 40% DM, dry enough to ensile immediately with minimal losses.
Since the losses in the dairy system are reduced as has been described above, part of the protein that is present in freshly cut grass is no longer required for the cow and this protein can be fed to pigs and poultry. The juice that is separated from the press cake during the process contains soluble protein which is not rumen resistant and therefore finds a much better application in monogastric animals.
Biorefinery effect on Nitrogen use efficiency and other environmental parameters
Fig. 5 shows a comparison of a traditional dairy system where 50% (or 25%) of the grass is ensiled, coupled with a pig rearing system and includes an integrated grass biorefinery system where 50% (or 25%) of the grass is biorefined. As a result of cultivation of compound feeds for the cows and for pigs, nitrogen fertilizer is lost on the field, therefore the outer system boundaries need 466kg and 317 kg respectively of nitrogen per ha to produce 67 kg of animal protein. Including a biorefinery approach to 50% of the grassland, improves the overall nitrogen use efficiency of the farm in this scenario from 14% and 21%. If the fertilizer losses to grow the compound feeds are not included and only the nitrogen content of the compound feeds is applied, then the NUE rises from 19.6 to 23% as can be seen in the inner system boundaries in Fig. 5.
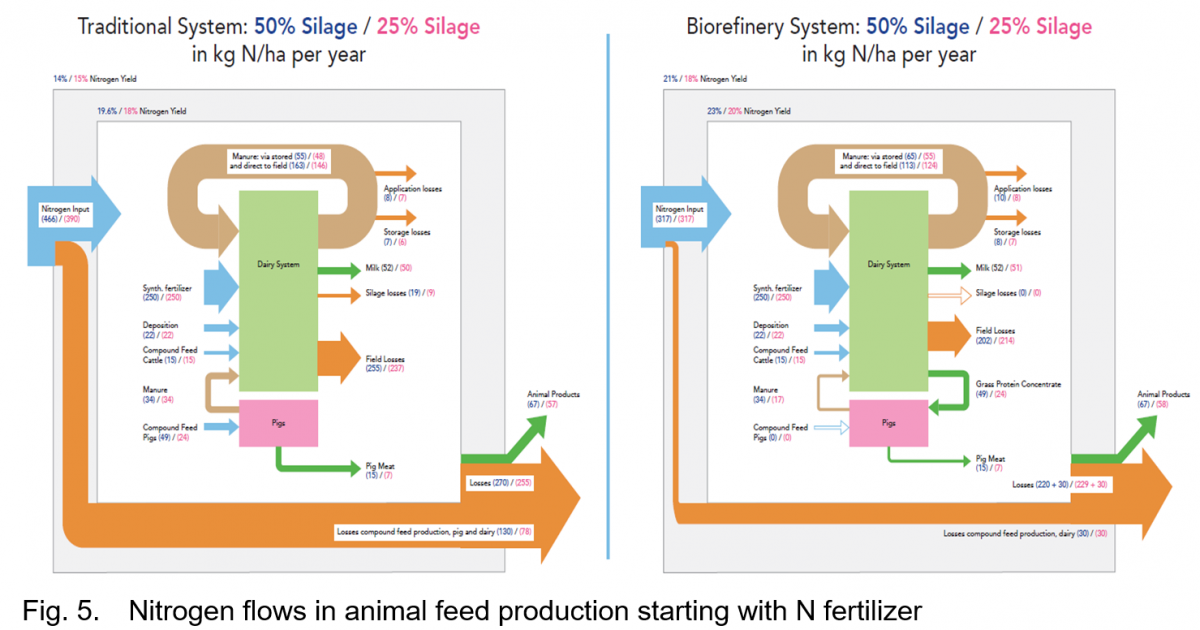
In a life cycle assessment (LCA) performed by Newcastle University, the environmental burdens of soymeal and leaf protein concentrate were compared. Greenhousgas emissions (GHG) and agricultural land use of both products were calculated for a simple biorefinery (no FOS nor minerals concentrates produced). (Tallentire et al.)

In the current technology, diesel is used to produce the 35 kWh electric energy and 55 kWh heat required to process one MT of fresh grass. In the future, renewable energy can be obtained from biogas and or from solar panels to meet these requirements. Furthermore, pinch technology can significantly reduce the energy requirements required for the coagulation of the protein. The operational profit is very dependant on local conditions
Economy
The capital cost for an 8 MT/hour equipment separating fresh grass into baled press cake, dried protein concentrate, liquid FOS concentrate and liquid mineral concentrate is estimated at approximately 1.8MUS$ under Dutch conditions. About half of the operating costs are associated with the raw materials, with labor and energy costs forming the other main costs. Income is spread almost equally over presscake, protein concentrate and the FOS including the minerals leading to an overall paybacktime of approximately 3 years in the Netherlands. Several business models can be envisaged like a cooperation of farmers who will fully operate the biorefinery. Another business model is rolled out by the Grassa company that buys the grass raw material, processes the grass and sells the 4 products in the market.
BROADER APPLICATION OF THE LEAVE BIOREFINERY TECHNOLOGY
Most green plant leaves can be processed by the Grassa technology. In the case of some raw materials, modifications will be required in the process, particularly with regards to the extruder press. A broadening of the technology application opens the opportunity to isolate proteins and other valuable components from leaves which are currently considered waste materials in agriculture or beyond. A recent study in Indonesia by Sari et al (submitted to BioFPR October 2020) showed that a protein shortfall of 9 M MT is expected with a more prosperous population of 300 million in Indonesia by 2035. Domestic (now wasted) raw materials such as cassava leaves, grasses, oil palm leaves and green rice leaves which can be obtained directly after the rice harvest, could supply 22-33 M MT of protein if biorefinery technology such as from Grassa becomes available. Currently these resources are left on the field where the protein is degraded and converted to ammonia and nitrate, part of which is leached out by rain or emitted to the atmosphere.
In the Netherlands, further examples exist of high-quality protein sources such as beet leaves, catch crop leaves and vegetable residues can form good sources of protein for monogastric animals.
Legumes as raw material for milk production can reduce the need for fertilizer production in factories using lots of energy
It has been demonstrated that Mixed Species Swards containing several leguminous plant species can produce equivalent or higher dry matter and protein yields as compared to traditional grass lands, with little or no nitrogen added. (Grace, et al. 2019). While in traditional grasslands some 40-50% of the fertilizersapplied are lost as ammonia or nitrate, legumes show losses of less than 5% since the nitrogen is taken up by the plant continuously after it is fixated by bacterial nodules that are located on the plant roots. Since the amount of Nitrogen in the cow’s manure is higher than required for the optimal growth of the mixed species, part of the Nitrogen can be removed from the cow’s cycle in the form of proteins for poultry and pigs as has been shown above, An additional step of ammonia stripping which involves trapping the ammonia in an acid like sulphuric acid can be applied to further reduce N after the cattle manure has been fermented in an anaerobic digestor to obtain biogas. This stripping technology has already been introduced successfully to the market by the Dutch company Byosis https://www.byosis.com/systems/byoflex(link is external).
In principle after this new value chain has been optimized in its entirety, it can be anticipated that a dairy farmer who now needs to purchase artificial fertilizers to grow grass to feed his cows, can now produce the same amount of milk as well as his own fertilizer in the form of ammonium sulphate, and can also produce protein for pigs on the very same hectare of meadow.
Even very small scale biorefinery systems can be economical in developing countries
In developing countries where fertilizers like N,P,K are expensive, the biorefinery approach combined with leguminous plants can offer various advantages: In those countries, the amount of fertilizers used currently, if any, is below 10 kg/ha/year. If this level could be enhanced to 50 kg/ha/y, then crop yields can be expected to raise by a factor of three. In the scenario that crops are biorefined after harvest and only proteins, carbohydrates and fats are taken for humans and/or animal feeds, the K, P and some N minerals that remain can be recycled for the next season of plant growth. Only the losses should be supplemented which will mainly be potassium as phosphate does not leach out significantly and Nitrogen can be supplemented if one uses a leguminous plant.
If one considers the full production chain including transportation of harvested crops to a processing unit but also the recycling of the minerals that are required back on agricultural fields, then certainly with crops of high moisture content and processes which may even add water to the process, operation on a small-scale mode can be very advantageous. (Bruins and Sanders, 2012). A small-scale Grassa biorefinery was installed in Fort Portal, Uganda in 2016 with a capacity of 100 kg fresh leaves per hour (Fig. 6). Plans have been developed to double this capacity in 2021 with an economically viable process. The anticipated products are indicated in Figure 6 as well.
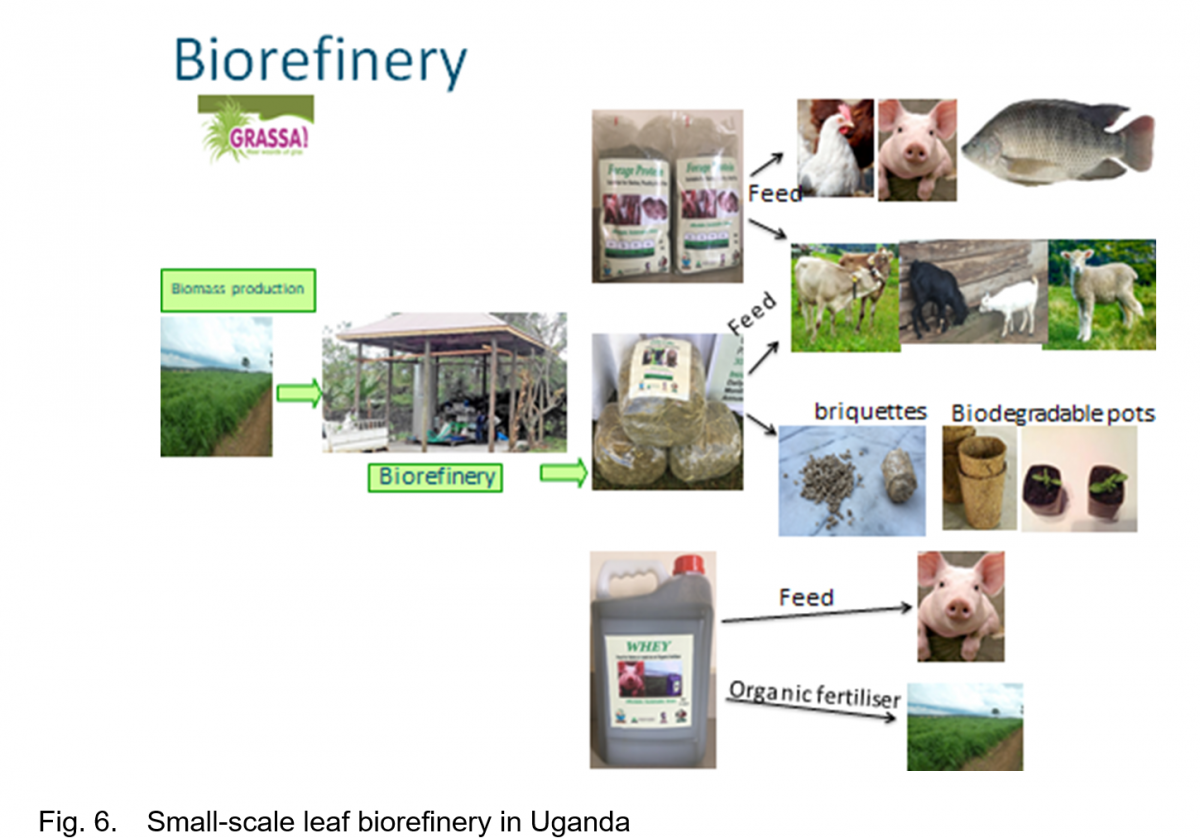
CONCLUSIONS, BIOREFINING OF LEAVES CONTRIBUTES TO STAY WITHIN PLANETARY BOUNDARIES
Tilman et al. (2011) anticipated that 110% more protein is required in 2050 as compared to 2005 to feed people and animals. Depending on improvements of technology and/or the transfer of technology to countries that do not have this technology yet, the demand for reactive nitrogen may grow from the current use of about 100 M MT to 210 M MT if we are to limit the need for land clearing with the consequent annual greenhouse gas emissions. Even with the best technology transfer and innovations there will be a requirement to clear about 500M ha resulting in 1.6 Gmt of CO2 eq emissions using 100 M MT of nitrogen fertilizer annually, or about 150 M ha if applying 210 M MT of nitrogen. This demonstrates that we must cross the Planetary boundaries severely unless we use agricultural land more efficiently and improve overall nitrogen use efficiency through the technology improvements that Tilman et al. describe. In addition, Mottet et al. (2017) show that nowadays some 2500 M ha of grasslands is in use for livestock production and that with modest improvements in feed use efficiencies we can prevent the need for further land use expansion.
In this paper we have shown that Biorefinery technology as well as inclusion of legumes in pastures, will enable improved feed use efficiency and will prevent the need for more landuse and reduce Nitrogen use as well. This increased efficiency is obtained globally by increasing field yields through recycling of fertilizers particularly in developing countries, combined with an optimization of grasslands globally by reducing the losses of grass during harvesting, reducing nitrogen input requirements of grasslands and by making better use of different types of protein that are better accessed after the biorefinery process. In addition to the considerable environmental benefits, agricultural incomes will also increase from such an approach and marginal land from which grass can be harvested will become a structural source of animal feed proteins.
REFERENCES
Bruins, M.E. and Sanders, J.P.M. 2012. Small‐scale processing of biomass for biorefinery. Biofpr, Wiley.40 (6) 2: 135-145.
Dijkstra, J., Reynolds, C.K., Kebreab, E., Bannink, A., Ellis, J.L., France, J. and Van Vuuren, A.M. 2013. Challenges in ruminant nutrition: towards minimal nitrogen losses in cattle. In: Oltjen J.W., Kebreab E., Lapierre H. (Eds.) Energy and protein metabolism and nutrition in sustainable animal production. Energy and protein metabolism and nutrition in sustainable animal production.134; 47-58. Wageningen Academic Publishers, Wageningen.
Department of Agriculture Food and the Marine.
Code of Good Agricultural Practice for reducing Ammonia Emissions from Agriculture. (https://www.agriculture.gov.ie/media/migration/ruralenvironment/ climatechange/CodeGoodAgriculturalPracticeReducingAmmoniaEmissions.pdf(link is external) ;accessed 29 September 2020).
European Soy monitor (https://www.idhsustainabletrade.com/uploaded/2019/04/ European-Soy-Monitor.pdf(link is external) ;accessed 29 September 2020).
Gaffey, J., and Sanders, J.P.M. 2018. Biorefineries and Small-scale Bioeconomy Opportunities for the Agriculture Sector. (https://www.engineersireland. ie/Engineers-Journal/Electrical/biorefineries-and-small-scale-bioeconomy-opportunities-for-the-agriculture-sector(link is external); accessed 2October2020).
Grace, C., Boland, T.M., Sheridan, H., Brennan, E., Fritch, R. and Lynch, M.B. 2019. The effect of grazing versus cutting on dry matter production of multispecies and perennial ryegrass‐only swards. Grass and forage science. 74(3); 437-449.
Hassouna, M. and Eglin, T. Measuring emissions from livestock farming: greenhouse gases, ammonia
and nitrogen oxides (https://www6.inrae.fr/animal_emissions/content/download/ 3345/35207/version/1/file/Hassouna%26Eglin2015_MeasuringEmissionsLivestockFarming-RMT-ADEME_march2016_EN.pdf(link is external) ;accessed 29 September 2020).
Leip, A., Weiss, F., Lesschen, J.P. and Westhoek, H. 2013.The nitrogen footprint of food products in the European Union. Journal of Agricultural Sciences. 152; 20-33.
Lesschen, J.P. and Sanders, J.P.M. 2017. Protein and nitrogen flows and efficiencies, in Sustainable Protein Technology. Wageningen University and Research Report 1786.
Mottet, A., de Haan, C., Falcucci, A., Tempio, G., Opio, C. and Gerber, P.; Livestock. 2017. On our plates or eating at our table? A new analysis of the feed/food debate. Global Food security. 14;1-8.
Pijlman, J., Koopmans, S., de Haan, G., Lenssinck, F., van Houwelingen, K.M., Sanders, J.P.M., Deru, J.G.C. and Erisman, J.W. 2018. Effect of feeding the grass fibrous fraction obtained from biorefinery on N and P utilisation of dairy cows. p. 431-433. In: 20th Nitrogen Workshop: "Coupling C-N-P-S cycles". Rennes, France. 25-27 June 2018.
Rockström, J., Steffen, W., Noone, K. and Persson, Å. 2009. Planetary boundaries: exploring the safe operating space for humanity. Ecology and Society. 14(2); 32(link is external).
Steffen, W., Richardson, K., Rockström, J., Cornell, S. E., Fetzer, I., Bennett, E.M., Biggs, R., Carpenter, S.R., de Vries, W., de Wit, C.A., Folke, C., Gerten, D., Heinke, J., Mace, G.M., Persson, L. M., Ramanathan, V., Reyers, B. and Sörlin, S. 2015. Planetary boundaries: Guiding human development on a changing planet. Science. 347(6223)1259855.
Tallentire, C.V., MacKenzie, S.G. and Kyriazakis, I. 2018. Can novel ingredients replace soybeans and reduce the environmental burdens of European livestock systems in the future? Journal of Cleaner Production. 187; 338-347.
Tilman, D., Balzer, C., Hill, J. and Befort, B.L. 2013. Global food demand and global intensification of agriculture. PNAS. December 13, 2011. 108 (50) 20260-20264.
Biorefinery for Increased Fertilizer and Land Use Efficiency and Better Incomes for Agriculture
ABSTRACT
The population of the world is ever-growing. It is expected to reach 10 billion in 2050. To feed all those people, significant improvements in our food production systems are required. Current agricultural practices have proven damaging to ecological systems worldwide, leading to greenhouse gas emissions, deforestation, loss of biodiversity and water pollution, among other problems. Moreover, two-thirds of agricultural land is currently used to feed livestock. We have already overstepped the Planetary Boundary as defined by Rockström et al for reactive nitrogen usage. Simply upscaling agricultural activities to meet the demands of the growing population is therefore not an option. Such an approach would lead to a Nitrogen fertilizer requirement which is three times higher than the world can sustain. We therefore need to become three times more efficient in using Nitrogen and consciously evaluate the way we produce food. New and disruptive innovations are needed to ensure a sustainable future for the next generations. This paper describes small-scale grass biorefinery technology which will contribute to solving three major issues: agricultural nitrogen emissions, the need for more agricultural land and the import of soy. Grass can be fully upgraded to the following components: (1) Refined grass: highly digestible grass which is high in fiber and rumen undegradable proteins, which is ideal as roughage for ruminants (dairy cattle, cattle, rabbits) or horses; (2) Grass protein concentrate: dried protein concentrate that is readily digestible with an optimal amino acid composition and which is an appropriate alternative to environmentally harmful soy meal in chicken, piglet, petfood or fish feeds; (3) FOS: Fructo Oligo Saccharides, a soluble fiber that acts as a prebiotic which improves digestion, stimulates the immune system, generally improves animal health and wellbeing and lowers the need for antibiotics; and (4) Mineral concentrate: which is rich in potassium and other minerals and in organic nitrogen which can serve as an organic, soluble, plant-based alternative for chemical or animal-derived fertilisers. The technology can be economically operated in grass rich countries at a scale of 8 MT of fresh grass per hour. In developing countries, it can be operated economically on a scale of 0.4 MT per hour. Both systems can also process green leaves of other plants which are not currently used to produce food or feeds: leaves of cassava, palm leaves, sugar beet leaves, green leaves of rice collected just after the rice harvest, etc. When grass derived from marginal land is included, we can increase the production of protein without increasing the land required for agricultural cropping. Since the grass biorefinery splits soluble and insoluble proteins, the first can be fed to cattle and the latter to pigs and poultry because in so doing the efficiency by which these proteins are used increases by 50%. This again means that one-third less land, and one-third less N, P, K fertilizers are required. As 50% more animal protein can be produced from the same hectare of grassland more economical value will be created which can be divided over the players in the chain: crop and grass farmers, biorefinery and animal farmers.
Keywords: Nitrogen Planetary Boundary, Biorefinery, Grass, Small scale, Protein
INTRODUCTION
The population of the world is ever-growing. It is expected to reach 10 billion in 2050. To feed all those people, significant improvements in our food production systems are required. Current agricultural practices, however, have proven damaging to ecological systems worldwide leading to greenhouse gas emissions, deforestation, loss of biodiversity and water pollution, among other things. Moreover, two-thirds of agricultural land is currently used for feeding livestock. Simply upscaling agricultural activities to meet the demands of the growing population is therefore not an option. We need to become more efficient and consciously evaluate the way we produce food. New and disruptive innovations are needed to ensure a sustainable future for the next generation.
The ecological capacity of our planet is coming under increasing pressure. The emission of greenhouse gases obviously contributes to global warming, however that is not the only problem, unfortunately. Johan Rockström and his team defined several ‘Planetary Boundaries’ in a 2009 model, which was updated in 2015 (See Fig. 1). This model visualises the severity of different threats to the planet. This research indicates that the level of nitrogen emissions may present an even bigger problem to our planet than the emission of greenhouse gases. This problem is largely rooted in agriculture and our unsustainable food and feed production systems. The use of nitrogen as a fertilizer and for chemical products has brought us enormous benefits in the past century. However, losses of fertilizer nitrogen and NOx from combustion processes to the environment lead to many side effects on human health, ecosystem health and the climate.
Agriculture is an important source of reactive nitrogen in the atmosphere and water bodies. Currently, humans produce 131 million MT of ammonia globally each year, which is about 50 million MT more than the planet can sustain. Around 75% of these emissions are estimated to originate from livestock farming. The consequences include soil acidification, loss of biodiversity and ‘algae blooms,’ resulting in anoxic zones in the oceans. Figure 2 provides a global representation of the areas with greatest excess nitrogen use per hectare. In the Netherlands, for example, the nitrogen crisis has already become a cause of major concerns in the past year, with at least 35% of all nitrogen losses to nature stemming from agricultural practices. One of the proposed solutions to this problem is to decrease the amount of livestock held by farmers, and although livestock is accountable for the majority of ammonia production through manure and urine wastes, half of the total Dutch cattle herd would need to be halved in order to reach acceptable emissions of nitrogen. This would, however, have serious consequences for thousands of farmers who rely on livestock herding for their livelihood and is also damaging to the Dutch economy. The same could be argued for many other countries whose economies depend partly on livestock farming and export of agricultural produce.
In Ireland, for example, there has been a mandatory ammonia emission target for agricultural businesses since 2010. From 2030 onwards, ammonia emissions must be reduced to 1% below 2005 levels by 2020 and 5% below 2005 levels. Agriculture is responsible for most of these emissions, and in recent years, Ireland’s ammonia emissions from agriculture have been increasing (Department of Agriculture, Food and the Marine). The Irish government has already proposed several methods for reducing ammonia emissions, among which include adapting livestock feeding strategies by extended grazing, maintaining the quality of crude protein when making silage or use of low-crude protein in animal feeds.
In France, studies on nitrogen emissions have been performed as well. As a result, livestock production has been identified as a major contributor to national ammonia, methane and nitrous oxide emissions. Farmers have been advised to take these emissions into account as part of efforts to mitigate climate change and improve air and water quality, when considering changes in farming practices such as feeds, types of livestock buildings, emission treatment, etc.(Hassouna and Eglin).
By using some 130 Mmt of nitrogen fertilizer in agriculture, worldwide, we have already overstepped the Planetary Boundary. With a population growing to 10 billion by 2050 which means we will eat more meat, this problem will become even much larger. Taking the efficiency of European protein-production for human food which requires 7 kg N input to obtain 1 kg N in food-protein to feed people with the required minimum of 50 grams per day, we will need an amount of Nitrogen fertilizer equivalent to 3 times the Planetary Boundary in 2050 (Table1). These numbers will be even larger when we realize that many western people consume 100 grams of protein or more. To stay within Planetary Boundaries the Nitrogen Use Efficiency (NUE) needs to be raised from 15% to over 35%, and the intake of protein should be reduced to 50 gram/person/day. While currently two-thirds of good agricultural land (1500Mha globally) is used for animal feed production, much of this feed should be produced on marginal lands to limit use of good agricultural land to direct human food production and in order to reduce further loss of biodiversity, another Planetary boundary under pressure.
SOLUTIONS TO STAY WITHIN PLANETARY BOUNDARIES
Halving the livestock herd in order to reduce nitrogen and ammonia emission levels would undoubtedly be a drastic measure, which might not be the most desirable way to go when considering the ever growing demand in, for example, meat and dairy products for food-grade proteins for a growing and more prosperous human population. Other measures such as more efficient feeding and development of alternative protein sources could prove to be an effective solution, without increasing demand for agricultural land or damage to rural economies.
This can be achieved by matching the amount and quality of nutrients consumed to that needed to meet the animals’ requirements and increasing the efficiency of utilization of the nutrients consumed. Currently, rations are often formulated with a ‘safety margin’ and based on mixing existing raw material streams within economic limits, which means the concentrations of nutrients are increased beyond the actual needs, sometimes by as much as 30 to 50%. This results in the production of excess nitrogen, phosphate and other residuals. Although composition can be good, the digestibility might be poor, which again leads to an oversupply of components in animal feeds. Reducing the excessive levels of nutrients and improving the efficiency of nutrient utilization, could therefore prove to be a simple, yet effective solution for the impending Global nitrogen crisis.
Matching the applied amount and quality of feeds can be achieved by adding essential amino acids to monogastric feeds to reduce the total amount of protein in the diet. Even more powerful is the splitting or refining of different components present in the actual feed raw materials, in order that proteins, fats, carbohydrates, minerals, vitamins, water and other required components are provided in a tailormade way to the animal throughout its life. This biorefinery approach exemplified in this paper with a focusing on grass and other leafy materials can also be applied more widely for most raw materials now utilized as animal feeds. Obviously, there are costs and energy requirements associated with this approach, and these will be addressed in this paper as well. Aside from reducing Nitrogen and phosphate emissions, less agricultural land is required when the biorefinery approach is utilized. Other benefits such as improved biodiversity and a reduction in transport of soy and raw materials are observed. The amount and volume of manure with concomitant emissions of NH3 and methane will also be reduced.
To cope with the Planetary limitations holistically, land use should also be optimized with yield gaps closed, while land that is suitable for crops for human consumption used for that purpose, with marginal lands such as wetlands, used for animal feed production. In the event that fertilizers are used, these should be applied in a way that limits losses to the environment or to the deeper layers in the soil. Legumes, the group of plants that can fixate nitrogen from the air using bacterial nodules on their roots, generally have much lower losses of reactive nitrogen because this is produced close to the roots. Vegetable crops in the Netherlands often have a fertilization efficiency of less than 30% which can be improved by drop irrigation. Once proteins are formed, one should realize that their value is much higher than when these proteins would be used as nitrogen fertilizer, by leaving agricultural residues on the field containing this protein. The same reasoning holds for animal waste streams which may face more difficult reuse limitations for safety and hygiene reasons. It is inevitable that animal manure contains minerals. These can be recycled to agriculture if there is no excess of these minerals. In cases where mineral excesses exist, such as in the Netherlands, ammonia can be stripped from anaerobic digested manure with technology that has been developed e.g. by a Dutch company Byosis, an approach that is now in operation in various countries around the world.
Finally, we can adjust the need for reactive nitrogen which limits the amount of daily protein consumed to the minimal requirements of about 50 grams, while in many western countries, the consumption of protein is more than double per head of population.
In 2017, the EU used an estimated 34.4 million MT of soybeans, soymeal and soybean oil, approximately 12% of global soybean production (European Soy monitor). By far the biggest proportion of this soy (30.8 million) was used as soymeal for agricultural purposes. As soy grows in temperate, subtropical and tropical climates, its use is mostly dependent on imports. Most of its production is concentrated in North and South America and aside from China, the EU is its biggest customer. Currently, around 85% of the global soybean harvest is crushed, resulting in around 78.5% soymeal and 18.5% soybean oil. The oil is used for varying purposes, while virtually all soymeal is used in compound feeds. Soy’s global success can be explained by the fact that it is a low cost, storable and transportable protein, as a side product of soy oil. Soy has, consequently, been one of the most important drivers of regional economic growth in Argentina, Brazil and Paraguay. As a result, the soybean industry is also connected to a range of environmental and social sustainability issues in the producing countries. Rapidly growing amounts of landmass have been dedicated to soy cultivation in the past few decades, leading to deforestation (more than 792,000 square kilometres of rainforest have been lost since 1970 in Brazil alone) and conversion of natural ecosystems.
Six factors are essential to optimize NUE and landuse at the same time:
While in European husbandry the nitrogen inputs are seven times higher than the nitrogen that ends up as protein in animals and vegetative consumer products, the reduction of losses will improve the efficiency of the animal feed system (Leip, et al 2013) According to Lesschen and Sanders, the Nitrogen use efficiency can be improved by a factor two in the Netherlands by the year 2050 (Lesschen and Sanders). This will be accomplished by reducing ammonia emission from manure storage and stables, and growing catch crops in the same season directly after corn. Protein will be extracted from these catch crops by biorefinery technology. This biorefinery technology will be applied to grass as well. Biorefining the raw materials that nowadays are used as animal feeds, will not only improve the N use efficiency, but will also enable the use of feed components such as protein at the highest value, e.g. in pig or poultry while cattle will benefit less from such protein. Additional components with no value for animals can be used to substitute fossil resources or to improve fertilizer use efficiency. This will reduce overall greenhouse gas emissions.
In this paper we will introduce the biorefinery- process and the products that can be obtained from grass and from several other sources. Furthermore, we will report several animal feeding results obtained with the products from the biorefinery.
GRASS BIOREFINERY
The Dutch company, Grassa has developed a bio-refinery process that upgrades grass and vegetable green residue streams to new and high-quality components that can serve as compound feed ingredients. Although the refinery process is suited for processing various raw materials (e.g. grass, beet leaves or pea plants), Grassa will initially focus on grass, clover and vegetable wastes. For simplicity, we will simply refer to those raw materials as ‘grass’ throughout this application.
Grassa biorefinery products
Through biorefining, grass can be fully split into the following components:
With these products, Grassa’s serves as an economically viable solution with a sustainable business case which also enhances food security for humans and animals. Through the refinery process we enable the local production of proteins, which is good for rural development, in a sustainable and circular way and without any use of pesticides. Grassa’s products are a great source of locally produced and easily digestible proteins and fibers, which are in high demand in the strongly growing organic agricultural sector. In some places, the demand for proteins and fibers is so high that it can barely be met. Organic/biological animal farming in Germany, for example, requires the use of exclusively German-produced animal feed raw materials. Bio-refining of biological grass makes it possible to meet the ever-growing demands for fiber and protein.
In addition, bio-refinery provides an elegant solution for the reduction of nitrogen emissions in the form of ammonia. In the Netherlands, we can now obtain approximately 50% higher yield of animal proteins per hectare, which now produces 12,000 kgs of milk, through the biorefinery approach which opens the structure of grass and fractionates the proteins present.
Grassa Bio-refinery technologies
Grassa developed various technologies one on 2 MT/hour capacity (Fig. 3) that is mobile, but the larger capacities still can be transported to other locations but several lorries will be required. The process that uses different unit operations (Fig. 4) for refining grass, such as pressing, protein extraction, nano-filtration and reverse osmosis. The grass is crushed and pressed first, separating juice from the grass fibers. The following process comprises several steps. The refined grasspresscake is compressed and sealed airtight for the purpose of conservation. The juice contains part of the nutrients (proteins, carbohydrates and minerals) and is processed by extracting and concentrating the proteins. The fluid that remains is further processed and is separated in a sugar fraction, a mineral rich fraction and clean water. The bio-refinery installation is designed in a small to medium size for local or regional processing of biomass. Although the focus for deployment of this technology is currently on cultivated and natural grass, clover and vegetable waste streams, in the future practically all types of fresh green materials or leaves could be processed using these installations and technologies.
Grassa focuses on ‘green’ bio-refinery which means that they solely use separation technology in their processes. Grassa uses an extruder, coagulation machine and belt filter, protein separation and washing, nano-filtration, reverse osmosis and a protein dryer. As these technologies use no biological or chemical conversions, the products are minimally processed and all the dry matter ends up in one of the four products.
In the Netherlands the processing and operating capacity of the complete process is now 4 MT of fresh leaf material per hour. For 2021 the construction of an 8 MT/ hours biorefinery which can operate under economic conditions in the Netherlands is planned. The complete 8 MT installation can be transported but unlike earlier versions, should not be regarded as movable. The previous 2 MT/hour Demonstration unit which contained only the refined grass and protein concentrate production, is mounted on a trailer and is fully movable. In the summer of 2019 this installation went to Ireland for a six-week demonstration on five commerical farms as part of the Biorefinery Glas project.
For Grassa’s innovation, the concept is that contractors collect grass from farms with grasslands and horticulture with green residue streams whose biomass are traded for refinery in exchange for compensation. A local bio-refinery then converts the grass into four products that Grassa will sell commercially: Refined grass, Grass protein concentrate, FOS concentrate and mineral concentrate. Refined grass will be distributed as an end product to livestock farmers with dairy cows, horses or rabbits, Grass protein concentrate will be supplied in bulk to compound feed companies as a feed ingredient for poultry, pigs, fish and cows, FOS concentrate will be supplied in bulk as a feed ingredient for pigs and the mineral concentrate will be distributed towards greenhouse horticulture as a fertilizer product. In this way, raw material cycles can be closed, and no large transportation movements are needed for nutrients and minerals. The local need for soy imports will also decrease significantly. Moreover, each of the four components that the machines produce, hold considerable benefits in comparison to current products on the market for compound feeds.
Feeding refined grass presscake to cows
The refined grass that has been obtained as press cake in the Grassa process has been fed to cows during trials at Louis Bolk Institute in the Netherlands (Pijlman et al). During this trial control cows were fed with 13 kg silage (dry matter base) and 8.6 kg of concentrates while in the experimental diet, two-thirds of the silage was substituted by refined grass press cake. In Table 2, we see that the Nitrogen input has been reduced from 721 g to 605 g per cow per day but that the nitrogen in the milk is 162 g in both cases. Fecal and urine N excretion have been reduced, therefore. The same picture holds for phosphate.
Overall, the feed conversion efficiency increased from 1.39 to 1.49, an increase of 7%. The N and P efficiencies increased by 18% and 12% and if calculated back to the 8 kg of refined grass on the 21.6 kg of the total diet, these numbers would rise to 18%, 45% and 31%, respectively. Part of the explanation of these improvements can be attributed to the ratio of the components in the feeds, which are better adapted to the needs of the cow in the trial sample, whereas in the control sample N and P are given in excess of the cows needs. This can be more fully understood if one realizes that part of the protein fed to cows are degraded and converted to microbial protein. This conversion can be beneficial to the cow if the diet contains protein with low essential amino acids since the conversion to microbial proteins results in a much better essential amino acid composition. However, in the case of grass, the essential amino acid content is as high as in soy protein, then a higher proportion of resistancy is preferred since the microbial resynthesis of protein amounts to as much as a third of nitrogen input losses, due to inefficiencies of rumen microbial protein synthesis, the formation of rumen microbial nucleic acids, and the indigestibility of some of the formed microbial proteins. (Dijkstra, et al. 2013).
In addition, basic biochemistry teaches that for each peptide bond to be synthesized 7 ATP is required. This would require 0.5 kg of sugar for the peptide bonds in 1 kg of protein only, to be resynthesized from 1 kg of plant protein that is degraded in the rumen. Also the other components of the microorganisms also require energy to be (re)synthesized. This additional step in the food chain requires resources and should be considered in a way that is healthy for the animal and optimized for resource use efficiencies.
In the Netherlands, about 75% of grass is ensiled. Another advantage of the grass biorefinery as compared to traditional ensiling is that there are no losses during the ensiling process. In traditional ensiling, the farmer must first wait for two consecutive sunny days in order to dry the grass. Grass biorefinery can be done even during wet weather, so the farmer can better plan his activities and cut the grass at the time the quality of the grass is best. Unlike traditional ensiling, there are no losses in nitrogen during spreading and re-spreading of grass during drying and no losses from leaking grass juices or mall grass particles during the collection after drying. In good farming practices, losses of 15% are indicated but these can well be as high as 25% in some cases. The Nitrogen that is in the protein of this part of the grass will become available as fertilizer after that grass leaf has been degraded in the soil, but of this recycled nitrogen about 40% will be lost since the fertilization yield in grassland is about 60%. In the biorefinery process, the wet grass is immediately processed into grass press cake of approximately 40% DM, dry enough to ensile immediately with minimal losses.
Since the losses in the dairy system are reduced as has been described above, part of the protein that is present in freshly cut grass is no longer required for the cow and this protein can be fed to pigs and poultry. The juice that is separated from the press cake during the process contains soluble protein which is not rumen resistant and therefore finds a much better application in monogastric animals.
Biorefinery effect on Nitrogen use efficiency and other environmental parameters
Fig. 5 shows a comparison of a traditional dairy system where 50% (or 25%) of the grass is ensiled, coupled with a pig rearing system and includes an integrated grass biorefinery system where 50% (or 25%) of the grass is biorefined. As a result of cultivation of compound feeds for the cows and for pigs, nitrogen fertilizer is lost on the field, therefore the outer system boundaries need 466kg and 317 kg respectively of nitrogen per ha to produce 67 kg of animal protein. Including a biorefinery approach to 50% of the grassland, improves the overall nitrogen use efficiency of the farm in this scenario from 14% and 21%. If the fertilizer losses to grow the compound feeds are not included and only the nitrogen content of the compound feeds is applied, then the NUE rises from 19.6 to 23% as can be seen in the inner system boundaries in Fig. 5.
In a life cycle assessment (LCA) performed by Newcastle University, the environmental burdens of soymeal and leaf protein concentrate were compared. Greenhousgas emissions (GHG) and agricultural land use of both products were calculated for a simple biorefinery (no FOS nor minerals concentrates produced). (Tallentire et al.)
In the current technology, diesel is used to produce the 35 kWh electric energy and 55 kWh heat required to process one MT of fresh grass. In the future, renewable energy can be obtained from biogas and or from solar panels to meet these requirements. Furthermore, pinch technology can significantly reduce the energy requirements required for the coagulation of the protein. The operational profit is very dependant on local conditions
Economy
The capital cost for an 8 MT/hour equipment separating fresh grass into baled press cake, dried protein concentrate, liquid FOS concentrate and liquid mineral concentrate is estimated at approximately 1.8MUS$ under Dutch conditions. About half of the operating costs are associated with the raw materials, with labor and energy costs forming the other main costs. Income is spread almost equally over presscake, protein concentrate and the FOS including the minerals leading to an overall paybacktime of approximately 3 years in the Netherlands. Several business models can be envisaged like a cooperation of farmers who will fully operate the biorefinery. Another business model is rolled out by the Grassa company that buys the grass raw material, processes the grass and sells the 4 products in the market.
BROADER APPLICATION OF THE LEAVE BIOREFINERY TECHNOLOGY
Most green plant leaves can be processed by the Grassa technology. In the case of some raw materials, modifications will be required in the process, particularly with regards to the extruder press. A broadening of the technology application opens the opportunity to isolate proteins and other valuable components from leaves which are currently considered waste materials in agriculture or beyond. A recent study in Indonesia by Sari et al (submitted to BioFPR October 2020) showed that a protein shortfall of 9 M MT is expected with a more prosperous population of 300 million in Indonesia by 2035. Domestic (now wasted) raw materials such as cassava leaves, grasses, oil palm leaves and green rice leaves which can be obtained directly after the rice harvest, could supply 22-33 M MT of protein if biorefinery technology such as from Grassa becomes available. Currently these resources are left on the field where the protein is degraded and converted to ammonia and nitrate, part of which is leached out by rain or emitted to the atmosphere.
In the Netherlands, further examples exist of high-quality protein sources such as beet leaves, catch crop leaves and vegetable residues can form good sources of protein for monogastric animals.
Legumes as raw material for milk production can reduce the need for fertilizer production in factories using lots of energy
It has been demonstrated that Mixed Species Swards containing several leguminous plant species can produce equivalent or higher dry matter and protein yields as compared to traditional grass lands, with little or no nitrogen added. (Grace, et al. 2019). While in traditional grasslands some 40-50% of the fertilizersapplied are lost as ammonia or nitrate, legumes show losses of less than 5% since the nitrogen is taken up by the plant continuously after it is fixated by bacterial nodules that are located on the plant roots. Since the amount of Nitrogen in the cow’s manure is higher than required for the optimal growth of the mixed species, part of the Nitrogen can be removed from the cow’s cycle in the form of proteins for poultry and pigs as has been shown above, An additional step of ammonia stripping which involves trapping the ammonia in an acid like sulphuric acid can be applied to further reduce N after the cattle manure has been fermented in an anaerobic digestor to obtain biogas. This stripping technology has already been introduced successfully to the market by the Dutch company Byosis https://www.byosis.com/systems/byoflex(link is external).
In principle after this new value chain has been optimized in its entirety, it can be anticipated that a dairy farmer who now needs to purchase artificial fertilizers to grow grass to feed his cows, can now produce the same amount of milk as well as his own fertilizer in the form of ammonium sulphate, and can also produce protein for pigs on the very same hectare of meadow.
Even very small scale biorefinery systems can be economical in developing countries
In developing countries where fertilizers like N,P,K are expensive, the biorefinery approach combined with leguminous plants can offer various advantages: In those countries, the amount of fertilizers used currently, if any, is below 10 kg/ha/year. If this level could be enhanced to 50 kg/ha/y, then crop yields can be expected to raise by a factor of three. In the scenario that crops are biorefined after harvest and only proteins, carbohydrates and fats are taken for humans and/or animal feeds, the K, P and some N minerals that remain can be recycled for the next season of plant growth. Only the losses should be supplemented which will mainly be potassium as phosphate does not leach out significantly and Nitrogen can be supplemented if one uses a leguminous plant.
If one considers the full production chain including transportation of harvested crops to a processing unit but also the recycling of the minerals that are required back on agricultural fields, then certainly with crops of high moisture content and processes which may even add water to the process, operation on a small-scale mode can be very advantageous. (Bruins and Sanders, 2012). A small-scale Grassa biorefinery was installed in Fort Portal, Uganda in 2016 with a capacity of 100 kg fresh leaves per hour (Fig. 6). Plans have been developed to double this capacity in 2021 with an economically viable process. The anticipated products are indicated in Figure 6 as well.
CONCLUSIONS, BIOREFINING OF LEAVES CONTRIBUTES TO STAY WITHIN PLANETARY BOUNDARIES
Tilman et al. (2011) anticipated that 110% more protein is required in 2050 as compared to 2005 to feed people and animals. Depending on improvements of technology and/or the transfer of technology to countries that do not have this technology yet, the demand for reactive nitrogen may grow from the current use of about 100 M MT to 210 M MT if we are to limit the need for land clearing with the consequent annual greenhouse gas emissions. Even with the best technology transfer and innovations there will be a requirement to clear about 500M ha resulting in 1.6 Gmt of CO2 eq emissions using 100 M MT of nitrogen fertilizer annually, or about 150 M ha if applying 210 M MT of nitrogen. This demonstrates that we must cross the Planetary boundaries severely unless we use agricultural land more efficiently and improve overall nitrogen use efficiency through the technology improvements that Tilman et al. describe. In addition, Mottet et al. (2017) show that nowadays some 2500 M ha of grasslands is in use for livestock production and that with modest improvements in feed use efficiencies we can prevent the need for further land use expansion.
In this paper we have shown that Biorefinery technology as well as inclusion of legumes in pastures, will enable improved feed use efficiency and will prevent the need for more landuse and reduce Nitrogen use as well. This increased efficiency is obtained globally by increasing field yields through recycling of fertilizers particularly in developing countries, combined with an optimization of grasslands globally by reducing the losses of grass during harvesting, reducing nitrogen input requirements of grasslands and by making better use of different types of protein that are better accessed after the biorefinery process. In addition to the considerable environmental benefits, agricultural incomes will also increase from such an approach and marginal land from which grass can be harvested will become a structural source of animal feed proteins.
REFERENCES
Bruins, M.E. and Sanders, J.P.M. 2012. Small‐scale processing of biomass for biorefinery. Biofpr, Wiley.40 (6) 2: 135-145.
Dijkstra, J., Reynolds, C.K., Kebreab, E., Bannink, A., Ellis, J.L., France, J. and Van Vuuren, A.M. 2013. Challenges in ruminant nutrition: towards minimal nitrogen losses in cattle. In: Oltjen J.W., Kebreab E., Lapierre H. (Eds.) Energy and protein metabolism and nutrition in sustainable animal production. Energy and protein metabolism and nutrition in sustainable animal production.134; 47-58. Wageningen Academic Publishers, Wageningen.
Department of Agriculture Food and the Marine.
Code of Good Agricultural Practice for reducing Ammonia Emissions from Agriculture. (https://www.agriculture.gov.ie/media/migration/ruralenvironment/ climatechange/CodeGoodAgriculturalPracticeReducingAmmoniaEmissions.pdf(link is external) ;accessed 29 September 2020).
European Soy monitor (https://www.idhsustainabletrade.com/uploaded/2019/04/ European-Soy-Monitor.pdf(link is external) ;accessed 29 September 2020).
Gaffey, J., and Sanders, J.P.M. 2018. Biorefineries and Small-scale Bioeconomy Opportunities for the Agriculture Sector. (https://www.engineersireland. ie/Engineers-Journal/Electrical/biorefineries-and-small-scale-bioeconomy-opportunities-for-the-agriculture-sector(link is external); accessed 2October2020).
Grace, C., Boland, T.M., Sheridan, H., Brennan, E., Fritch, R. and Lynch, M.B. 2019. The effect of grazing versus cutting on dry matter production of multispecies and perennial ryegrass‐only swards. Grass and forage science. 74(3); 437-449.
Hassouna, M. and Eglin, T. Measuring emissions from livestock farming: greenhouse gases, ammonia
and nitrogen oxides (https://www6.inrae.fr/animal_emissions/content/download/ 3345/35207/version/1/file/Hassouna%26Eglin2015_MeasuringEmissionsLivestockFarming-RMT-ADEME_march2016_EN.pdf(link is external) ;accessed 29 September 2020).
Leip, A., Weiss, F., Lesschen, J.P. and Westhoek, H. 2013.The nitrogen footprint of food products in the European Union. Journal of Agricultural Sciences. 152; 20-33.
Lesschen, J.P. and Sanders, J.P.M. 2017. Protein and nitrogen flows and efficiencies, in Sustainable Protein Technology. Wageningen University and Research Report 1786.
Mottet, A., de Haan, C., Falcucci, A., Tempio, G., Opio, C. and Gerber, P.; Livestock. 2017. On our plates or eating at our table? A new analysis of the feed/food debate. Global Food security. 14;1-8.
Pijlman, J., Koopmans, S., de Haan, G., Lenssinck, F., van Houwelingen, K.M., Sanders, J.P.M., Deru, J.G.C. and Erisman, J.W. 2018. Effect of feeding the grass fibrous fraction obtained from biorefinery on N and P utilisation of dairy cows. p. 431-433. In: 20th Nitrogen Workshop: "Coupling C-N-P-S cycles". Rennes, France. 25-27 June 2018.
Rockström, J., Steffen, W., Noone, K. and Persson, Å. 2009. Planetary boundaries: exploring the safe operating space for humanity. Ecology and Society. 14(2); 32(link is external).
Steffen, W., Richardson, K., Rockström, J., Cornell, S. E., Fetzer, I., Bennett, E.M., Biggs, R., Carpenter, S.R., de Vries, W., de Wit, C.A., Folke, C., Gerten, D., Heinke, J., Mace, G.M., Persson, L. M., Ramanathan, V., Reyers, B. and Sörlin, S. 2015. Planetary boundaries: Guiding human development on a changing planet. Science. 347(6223)1259855.
Tallentire, C.V., MacKenzie, S.G. and Kyriazakis, I. 2018. Can novel ingredients replace soybeans and reduce the environmental burdens of European livestock systems in the future? Journal of Cleaner Production. 187; 338-347.
Tilman, D., Balzer, C., Hill, J. and Befort, B.L. 2013. Global food demand and global intensification of agriculture. PNAS. December 13, 2011. 108 (50) 20260-20264.