ABSTRACT
Precision agriculture is increasingly becoming part of contemporary agriculture in smallholder farming systems in the developing world. It contributes to improving crop productivity and hence attainment of food security and improved income for the farmers, while reducing leakages to the environment and thus foster environmental sustainability. This paper focuses on precision nutrient management in rice based cropping system using scientific principles of site-specific nutrient management (SSNM). The SSNM principles were developed to determine field specific N, P and K requirements for rice crop through partnerships of the International Rice Research Institute (IRRI) with organizations across Asia. Using SSNM principles, a web based ICT decision support tool was developed to provide field- and season-specific nutrient management recommendations to rice farmers; Rice Crop Manager (RCM; http://webapps.irri.org/ph/rcm/). RCM is a cloud-based decision support tool that calculates nutrient requirements based on farmers’ responses to 25-30 easy to answer questions on farm practices and field conditions. It was designed to address heterogeneity, which can be high within small distances in rice growing environments, dominated by smallholder farmers. RCM was released for rice based cropping systems in the Philippines, India and Bangladesh and has been shown to improve rice yields with positive added net benefit for farmers. Thus, with minimum modification and localisation, RCM has potential to be used in other rice growing environments, within and outside Asia.
Keywords: precision agriculture, nutrient management, ICT, rice production
INTRODUCTION
Site-specific crop management, or precision agriculture, is increasingly becoming an important component of modern agriculture with the main aim of increasing crop productivity while optimizing resources, reducing production costs and fostering environmental sustainability, and thus improve efficiency of land use (Gebbers and Adamchuk, 2010). Precision agriculture was initiated in the 1980s mainly to improve fertilizer application by varying the rates within the field in order to cater for spatial variability, owing to improved investigation methods such as soil surveys and mapping, aerial photography, etc. (Robert, 2002). It can be defined as “the application of modern information technologies to provide, process and analyze multisource data of high spatial and temporal resolution for decision making and operations in the management of crop production” (National Research Council, 1997). Precision agriculture is continuously evolving, increasingly utilizing multi-dimensional and multi-disciplinary information to equip decision makers with the ability to choose management options for improving agricultural production systems. With the evolution in technological advances, and the development of decision support tools, precision agriculture has also evolved, aiding farmers in decision making on crop management practices. Accurate yield estimation of crops, using historical information or predictive tools, is vital in order to develop optimized crop management practices. Although much of precision agriculture has been practiced on large farms linked with high-tech agriculture in developed countries, it is increasingly gaining traction in developing countries and on small farms. It can be considered as another revolution, following after the Green Revolution.
In Asia, rice (Oryza sativa L.) production benefited from the Green Revolution, which was introduced in the 1960s; arguably represents the most dramatic shift in agricultural practices in human history. Food crop production for cereal crops increased significantly due to a combination of factors; vis-à-vis adoption of improved varieties that were semi-dwarf, short duration and highly responsive to fertilizers, increased access to irrigation, and use of fertilizers and chemicals (Pingali, 2012). Increased use of fertilizers, particularly N, significantly improved crop yields globally, including rice (Lu and Tian, 2017). Rice production became intensified, with continuous production of two to three crops annually. This was important for the attainment of food security considering that rice is the staple food for many low- and middle-income countries constituting about half the global population. About 90% of the global rice is produced in Asia and that is also where most of it is consumed, but there is increasing demand in sub-Saharan Africa where dietary preferences are shifting from the traditional coarse grains owing to urbanization and changing family occupational structure. Rice provides about 25% of the global human energy consumption per capita and about 16% of protein (GRiSP, 2013).
Rice intensification led to an increase in fertilizer use, for example in Asia, it increased from 13 Mt in 1950 to 110 Mt in 1984, largely because of the striking yield increases and the immediate economic gains to the farmers with the use of fertilizers on nutrient responsive varieties with increased water access. However, this led to overuse of fertilizers, particularly nitrogen fertilizers, which was associated with a decline in yield responses to additional fertilizers and reduced fertilizer use eficiencies. Farmers in Asia often apply large quantities of N fertilizers, often in excess of plant N requirements, despite differences in crop performance and potential yields. By the 1990s, recovery efficiencies on N fertilizer were only about 30-50%, indicating losses to the environment of up to 70% of the applied N fertilizer (Dobermann, 2000), associated with pollution of waterways. This inefficient use of fertilizers has resulted in unprofitable farming, given that fertilizer is the second largest expense in rice production after labor (Pampolino et al., 2007).
Nutrient management plays an important role in closing the rice yield gap in Asia where yields obtained have on average been 65% of the genetic and climatic potential yield, despite being the largest global rice producer. Fertilizer management is an important component for sustainable rice production, for example in China farmers use excessive amounts of N fertilizer, with an average of 193 kg N ha-1 in 2006 (Heffer, 2009) yet the average yield was less that 6.5 t ha-1. On the other hand in Cambodia, in addition to low native soil fertility, high spatial and temporal variability affects rice yields yet farmers apply blanket fertilizer amounts (Seng et al., 2001). With the increasing global food demand caused by a growing population, which also causes an increasing pressure on land in the face of a changing and variable climate, options are needed to increase productivity per unit area while fostering environmental sustainability. Given the current trends of urban expansion into agricultural land, innovative solutions are need to increase crop production and profitability without expansion of land to new areas though sustainable intensification is (Pretty et al., 2011).
Site-Specific Nutrient Management
With the need to address the challenges of the declining rice yields observed in some long-term experiments in Asia and low nutrient use efficiencies (Cassman et al., 1995), the International Rice Research Institute (IRRI) in collaboration with partners across Asia developed and tested the site-specific nutrient management (SSNM) approach in the 1990’s (Dobermann et al., 2002). This approach is a form of precision nutrient management based on scientific principles and aims to improve yield within existing fields without expanding to new areas while enhancing nutrient use efficiency and protecting the environment, and thus sustainable intensification of rice cropping systems. Rice fields in Asia are usually small (often about one hectare or less) with high spatial variability with respect to nutrient status, use of varieties and crop management practices by farmers. This has resulted in variable rice performance, which is not addressed with traditional nutrient management practices of blanket/uniform fertilizer application across large areas. Blanket recommendations ignore variations across fields in supply of essential nutrients from the soil (Dobermann et al., 2002) and variations in crop management practices, which can influence the needs of the rice crop for fertilizer and the yields obtained in farmers’ fields .The SSNM approach takes into consideration the high spatial variability observed in smallholder farming systems in Asia, to ensure efficient utilization of indigenous nutrients and then supplying adequate nutrients needed to meet the requirements for a given target yield, while avoiding leakage to the environment.
Consequently, nutrient management in smallholder rice production systems need to be tailored to suit the conditions of different farms and seasons. Opportunities exist to increase the effectiveness of nutrient use and thus income for rice farmers through precision nutrient management. The management of fertilizers can be particularly critical for profitable rice farming in Asia because the emerging energy crisis and rising fuel prices have caused an increase in the cost of rice cultivation. Fertilizers must be applied at the right times and rates, using the right type for efficiently increasing yield per unit of nutrient applied.
The SSNM approach, a dynamic, field- and season-specific nutrient management system, was developed to calculate field-specific requirements for fertilizer N, P and K for cereal crops based on scientific principles (Dobermann et al., 2004). It was initially developed for rice but has been applied to wheat (Khurana et al., 2008) and maize (Witt et al., 2009). The approach was refined through years of research and in evaluation trials it was shown to increase rice yield and income (Dobermann et al., 2004). A recent study in India showed greater crop yields with SSNM compared to the farmer practice (Sharma et al., 2019), which is often based on blanket recommendations and in some cases, overuse of fertilizers. Much of the research on SSNM was done on favourable environments, mostly irrigated lowlands. Nonetheless, rice yields were also greater with SSNM than farmer practice under rainfed lowland conditions in the Philippines, India and Thailand (Banayo et al., 2018; Haefele and Konboon, 2009). However, the yield advantage was smaller compared to irrigated ecosystems. In some cases, both irrigated and rainfed, lower amounts of fertilizer are used with SSNM but still with greater yields than farmer practice. This can be attributed to the right time of application, particularly for N fertilizer, where N fertilizer timing coincides with crop demand, resulting in greater agronomic efficiency and hence greater utilization effeciciency of the applied N fertilizer.
Decision support tools
While the positive benefits of SSNM compared to the farmer practice, or local recommendation were demonstrated in different environments across different countries in Asia, the uptake of the SSNM recommendations remained low. Research partners linked with extension workers to extend the technology in different sites, but still the uptake remained low. This was largely because the SSNM approach is knowledge intensive and can not be easily used by extension to disseminate nutrient management recommendations to farmers. Using the principles of SSNM, IRRI developed and released an ICT decision support tool, Rice Crop Manager (RCM; http://cropmanager.irri.org) in the Philippines (Buresh et al., 2019), India (Sharma et al., 2019), Bangladesh, Indonesia and Vietnam to give field-specific fertilizer recommendations to individual smallholder farmers. It is a web-based decision support tool that that can be accessed using computers, tablets or smart phones connected to the internet. It is used to calculate field-specific nutrient management recommendations using information provided by a farmer through an interview. The farmer is asked 25-30 easy to answer questions about their field condition and crop management. At the end of the questionnaire Rice Crop Manager automatically calculates and gives easy to follow nutrient and crop management recommendations that can be printed on one page before the start of the season. RCM has been widely evaluated in the Philippines and has led to an increase in rice yield and income on average by 400 kg and $ 100 (PhP 6000) ha-1 season-1 over nine seasons on more than 1000 sites (Figure 1). In India the use of RCM resulted in positive rice and wheat yield gain with greater added net benefit when compared to the farmer practice (Table 1). RCM has potential to be released in other countries in the region including Cambodia, Myanmar, Sri Lanka, Thailand, with possibilities to expand to Africa. Its use can increase rice productivity to meet the challenges of rising demand for the commodity driven by population growth, while protecting the environment and contributing to attaining Sustainable Rice Platform (SRP) standards.
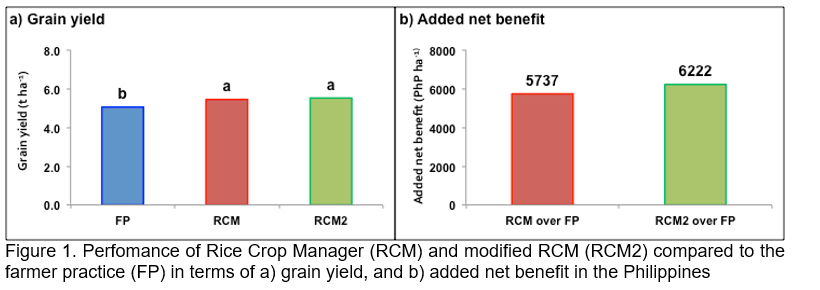
Table 1: Rice and wheat yield gain and added net benefit obtained with Rice Crop Manager (RCM) compared to the farmer practice in two states in India, Odisha and Bihar
Parameter
|
Unit
|
Effect of RCM (RCM-FP)
|
|
|
Odisha- Kharif Rice
|
|
Odisha-Rabi Rice
|
|
Bihar- Rice
|
|
Bihar- Wheat
|
|
Grain Yield
|
t ha-1
|
+0.8
|
*
|
+0.5
|
*
|
+0.7
|
*
|
+0.3
|
*
|
Net added Benefit
|
USD ha-1
|
155
|
*
|
104
|
*
|
147
|
*
|
63
|
*
|
* Significant at 0.05 level
Challenges
The increasing availability of ICT tools to provide accurate, timely and relevant crop management recommendations to farmers has been seen as an option for wider dissemination of information. While most farmers have access to mobile phones, only few have smart phones that can access the internet. Additionally, internet avilability and stability in rural areas, where many smallholder farmers are located, is generally poor and thus limiting the access to information. To improve access, RCM in the offline mode should be developed so that its use can be extended to areas with limited internet access.
As agriculture is increasingly being affected by climate change, changing and increasing insect pest and diseases, deteriorating natural resources and environmental pollution, there arises a need to equip these decision tools with updated and dynamic information which can cater to new generation agricultural problems. Fortunately there have been many new developments in soil and related subjects, which can increase the robustness of these tools and make them more dynamic. Improvement in precision of weather forecast systems, wherein one can have the information on the weather (rainfall, temperature, relative humidity, etc.) that is going to affect your crop in the coming days has potential to reduce the impact of adverse weather events. This will occur because the advance notice will allow decision makers the opportunity to implement plans to minimize the impact of adverse events and find opportunities within favorable events. Integration of weather information with other environmental information such as crop variety, soil type, soil maps, soil fertility, soil moisture and long-term drought conditions, can maximize the value of prediction information for decision-making process and will help farmers and agribusinesses utilize the information more effectively in decision-making.
The progress made in GIS and remote sensing has led to access to more accurate and timely information in the form of maps, statistics, and reports, crop production areas, crop health, crop losses due to natural calamities, and assessment and extent of pest damage. For example, IRRI developed Philippine Rice Information System (PRiSM) which uses satelite imagery to assess mid-season crop performance during the season and predict end of season yield. While RCM provides pre-season crop management recommendations using predictive and historical information, the actual performance in the field can be influenced by weather, pests, diseases and others. PRiSM can potentially be used to provide information that can be used to adjust RCM recommendations based on actual performance. A logical framework for integration and interface of the latest scientific developments in improved and resilient germplasm, improved and best crop and nutrient management, GIS and remote sensing, and weather forecasting can help to develop a robust and dynamic tools to guide the farmers with better and real time decision making. These tools have potential to revolutionize agriculture in the developing world.
REFERENCES
Banayo, N. P. M. C., Haefele, S., Desamero, N. V., and Kato, Y. (2018). On-farm assessment of site-specific nutrient management for rainfed lowland rice in the Philippines. Field Crops Research 220, 88-96.
Buresh, R. J., Laureles, E., Dela Torre, J. C., Samson, M., Guerra, M., Sinohin, P. J., and Castillo, R. (2019). Site-specific nutrient management for rice in the Philippines: Calculation of field-specific fertilizer requirements by Rice Crop Manager (under review). Field Crops Research.
Cassman, K. G., De Datta, S., Olk, D., Alcantara, J., Samson, M., Descalsota, J., and Dizon, M. (1995). Yield decline and the nitrogen economy of long-term experiments on continuous, irrigated rice systems in the tropics. Soil management: Experimental basis for sustainability and environmental quality, 181-222.
Dobermann, A. (2000). Future intensification of irrigated rice systems. In: Sheehy, J.E., Mitchell, P.L., Hardy, B. Eds.), Redesigning Rice Photosynthesis to Increase Yield. Interna- tional Rice Research Institute/Elsevier, Makati City, Philip- pines/Amsterdam, pp. 229-247.
Dobermann, A., Witt, C., and Dawe, D. (2004). Increasing the productivity of intensive rice systems through site-specific nutrient management. Science Publishers, Inc., Enfield, NH, USA and Int. Rice Res. Inst., Los Baños, Philippines.
Dobermann, A., Witt, C., Dawe, D., Abdulrachman, S., Gines, H., Nagarajan, R., Satawathananont, S., Son, T., Tan, P., and Wang, G. (2002). Site-specific nutrient management for intensive rice cropping systems in Asia. Field Crops Research 74, 37-66.
Gebbers, R., and Adamchuk, V. I. (2010). Precision agriculture and food security. Science 327, 828-831.
GRiSP, G. R. S. P. (2013). Rice almanac, 4th edition. Los Baños (Philippines). International Rice Research Institute. 283 p.
Haefele, S., and Konboon, Y. (2009). Nutrient management for rainfed lowland rice in northeast Thailand. Field Crops Research 114, 374-385.
Heffer, P. (2009). Assessment of fertilizer use by crop at the global level. International Fertilizer Industry Association, Paris, www. fertilizer. org/ifa/Home-Page/LIBRARY/Publication-database. html/Assessment-of-Fertilizer-Use-by-Crop-at-the-Global-Level-2006-07-2007-08. html2.
Khurana, H. S., Phillips, S. B., Alley, M. M., Dobermann, A., Sidhu, A. S., and Peng, S. (2008). Agronomic and economic evaluation of site-specific nutrient management for irrigated wheat in northwest India. Nutrient Cycling in Agroecosystems 82, 15-31.
Lu, C., and Tian, H. (2017). Global nitrogen and phosphorus fertilizer use for agriculture production in the past half century: shifted hot spots and nutrient imbalance. Ecology, Evolution and Organismal Biology Publications. 348.
National Research Council, N. (1997). Precision Agriculture in the 21st Century: Geospatial and Information Technologies in Crop Management. Washington, DC: The National Academies Press. https://doi.org/10.17226/5491.
Pampolino, M. F., Manguiat, I. J., Ramanathan, S., Gines, H., Tan, P., Chi, T., Rajendran, R., and Buresh, R. (2007). Environmental impact and economic benefits of site-specific nutrient management (SSNM) in irrigated rice systems. Agricultural Systems 93, 1-24.
Pingali, P. L. (2012). Green revolution: impacts, limits, and the path ahead. Proceedings of the National Academy of Sciences 109, 12302-12308.
Pretty, J., Toulmin, C., and Williams, S. (2011). Sustainable intensification in African agriculture. International journal of agricultural sustainability 9, 5-24.
Robert, P. C. (2002). Precision agriculture: a challenge for crop nutrition management. Plant and Soil 247, 143-149.
Seng, V., Ros, C., Bell, R., White, P., and Hin, S. (2001). Nutrient requirements of rainfed lowland rice in Cambodia. In: Fukai, S. and Basnayake, J., (eds.) Increased Lowland Rice Production in the Mekong Region; proceedings of an international workshop held in Vientiane, Laos, 30 Oct - 1 Nov. ACIAR, Canberra, pp. 169-178.
Sharma, S., Panneerselvam, P., Castillo, R., Manohar, S., Raj, R., Ravi, V., and Buresh, R. J. (2019). Web-based tool for calculating field-specific nutrient management for rice in India. Nutrient Cycling in Agroecosystems 113, 21-33.
Witt, C., Pasuquin, J., Pampolino, M., Buresh, R., and Dobermann, A. (2009). A manual for the development and participatory evaluation of sitespecific nutrient management for maize in tropical, favorable environments. International Plant Nutrition Institute, Penang. Dr. Timsina is shown visiting a maize field.
Precision Agriculture in Food Production: Nutrient Management
ABSTRACT
Precision agriculture is increasingly becoming part of contemporary agriculture in smallholder farming systems in the developing world. It contributes to improving crop productivity and hence attainment of food security and improved income for the farmers, while reducing leakages to the environment and thus foster environmental sustainability. This paper focuses on precision nutrient management in rice based cropping system using scientific principles of site-specific nutrient management (SSNM). The SSNM principles were developed to determine field specific N, P and K requirements for rice crop through partnerships of the International Rice Research Institute (IRRI) with organizations across Asia. Using SSNM principles, a web based ICT decision support tool was developed to provide field- and season-specific nutrient management recommendations to rice farmers; Rice Crop Manager (RCM; http://webapps.irri.org/ph/rcm/). RCM is a cloud-based decision support tool that calculates nutrient requirements based on farmers’ responses to 25-30 easy to answer questions on farm practices and field conditions. It was designed to address heterogeneity, which can be high within small distances in rice growing environments, dominated by smallholder farmers. RCM was released for rice based cropping systems in the Philippines, India and Bangladesh and has been shown to improve rice yields with positive added net benefit for farmers. Thus, with minimum modification and localisation, RCM has potential to be used in other rice growing environments, within and outside Asia.
Keywords: precision agriculture, nutrient management, ICT, rice production
INTRODUCTION
Site-specific crop management, or precision agriculture, is increasingly becoming an important component of modern agriculture with the main aim of increasing crop productivity while optimizing resources, reducing production costs and fostering environmental sustainability, and thus improve efficiency of land use (Gebbers and Adamchuk, 2010). Precision agriculture was initiated in the 1980s mainly to improve fertilizer application by varying the rates within the field in order to cater for spatial variability, owing to improved investigation methods such as soil surveys and mapping, aerial photography, etc. (Robert, 2002). It can be defined as “the application of modern information technologies to provide, process and analyze multisource data of high spatial and temporal resolution for decision making and operations in the management of crop production” (National Research Council, 1997). Precision agriculture is continuously evolving, increasingly utilizing multi-dimensional and multi-disciplinary information to equip decision makers with the ability to choose management options for improving agricultural production systems. With the evolution in technological advances, and the development of decision support tools, precision agriculture has also evolved, aiding farmers in decision making on crop management practices. Accurate yield estimation of crops, using historical information or predictive tools, is vital in order to develop optimized crop management practices. Although much of precision agriculture has been practiced on large farms linked with high-tech agriculture in developed countries, it is increasingly gaining traction in developing countries and on small farms. It can be considered as another revolution, following after the Green Revolution.
In Asia, rice (Oryza sativa L.) production benefited from the Green Revolution, which was introduced in the 1960s; arguably represents the most dramatic shift in agricultural practices in human history. Food crop production for cereal crops increased significantly due to a combination of factors; vis-à-vis adoption of improved varieties that were semi-dwarf, short duration and highly responsive to fertilizers, increased access to irrigation, and use of fertilizers and chemicals (Pingali, 2012). Increased use of fertilizers, particularly N, significantly improved crop yields globally, including rice (Lu and Tian, 2017). Rice production became intensified, with continuous production of two to three crops annually. This was important for the attainment of food security considering that rice is the staple food for many low- and middle-income countries constituting about half the global population. About 90% of the global rice is produced in Asia and that is also where most of it is consumed, but there is increasing demand in sub-Saharan Africa where dietary preferences are shifting from the traditional coarse grains owing to urbanization and changing family occupational structure. Rice provides about 25% of the global human energy consumption per capita and about 16% of protein (GRiSP, 2013).
Rice intensification led to an increase in fertilizer use, for example in Asia, it increased from 13 Mt in 1950 to 110 Mt in 1984, largely because of the striking yield increases and the immediate economic gains to the farmers with the use of fertilizers on nutrient responsive varieties with increased water access. However, this led to overuse of fertilizers, particularly nitrogen fertilizers, which was associated with a decline in yield responses to additional fertilizers and reduced fertilizer use eficiencies. Farmers in Asia often apply large quantities of N fertilizers, often in excess of plant N requirements, despite differences in crop performance and potential yields. By the 1990s, recovery efficiencies on N fertilizer were only about 30-50%, indicating losses to the environment of up to 70% of the applied N fertilizer (Dobermann, 2000), associated with pollution of waterways. This inefficient use of fertilizers has resulted in unprofitable farming, given that fertilizer is the second largest expense in rice production after labor (Pampolino et al., 2007).
Nutrient management plays an important role in closing the rice yield gap in Asia where yields obtained have on average been 65% of the genetic and climatic potential yield, despite being the largest global rice producer. Fertilizer management is an important component for sustainable rice production, for example in China farmers use excessive amounts of N fertilizer, with an average of 193 kg N ha-1 in 2006 (Heffer, 2009) yet the average yield was less that 6.5 t ha-1. On the other hand in Cambodia, in addition to low native soil fertility, high spatial and temporal variability affects rice yields yet farmers apply blanket fertilizer amounts (Seng et al., 2001). With the increasing global food demand caused by a growing population, which also causes an increasing pressure on land in the face of a changing and variable climate, options are needed to increase productivity per unit area while fostering environmental sustainability. Given the current trends of urban expansion into agricultural land, innovative solutions are need to increase crop production and profitability without expansion of land to new areas though sustainable intensification is (Pretty et al., 2011).
Site-Specific Nutrient Management
With the need to address the challenges of the declining rice yields observed in some long-term experiments in Asia and low nutrient use efficiencies (Cassman et al., 1995), the International Rice Research Institute (IRRI) in collaboration with partners across Asia developed and tested the site-specific nutrient management (SSNM) approach in the 1990’s (Dobermann et al., 2002). This approach is a form of precision nutrient management based on scientific principles and aims to improve yield within existing fields without expanding to new areas while enhancing nutrient use efficiency and protecting the environment, and thus sustainable intensification of rice cropping systems. Rice fields in Asia are usually small (often about one hectare or less) with high spatial variability with respect to nutrient status, use of varieties and crop management practices by farmers. This has resulted in variable rice performance, which is not addressed with traditional nutrient management practices of blanket/uniform fertilizer application across large areas. Blanket recommendations ignore variations across fields in supply of essential nutrients from the soil (Dobermann et al., 2002) and variations in crop management practices, which can influence the needs of the rice crop for fertilizer and the yields obtained in farmers’ fields .The SSNM approach takes into consideration the high spatial variability observed in smallholder farming systems in Asia, to ensure efficient utilization of indigenous nutrients and then supplying adequate nutrients needed to meet the requirements for a given target yield, while avoiding leakage to the environment.
Consequently, nutrient management in smallholder rice production systems need to be tailored to suit the conditions of different farms and seasons. Opportunities exist to increase the effectiveness of nutrient use and thus income for rice farmers through precision nutrient management. The management of fertilizers can be particularly critical for profitable rice farming in Asia because the emerging energy crisis and rising fuel prices have caused an increase in the cost of rice cultivation. Fertilizers must be applied at the right times and rates, using the right type for efficiently increasing yield per unit of nutrient applied.
The SSNM approach, a dynamic, field- and season-specific nutrient management system, was developed to calculate field-specific requirements for fertilizer N, P and K for cereal crops based on scientific principles (Dobermann et al., 2004). It was initially developed for rice but has been applied to wheat (Khurana et al., 2008) and maize (Witt et al., 2009). The approach was refined through years of research and in evaluation trials it was shown to increase rice yield and income (Dobermann et al., 2004). A recent study in India showed greater crop yields with SSNM compared to the farmer practice (Sharma et al., 2019), which is often based on blanket recommendations and in some cases, overuse of fertilizers. Much of the research on SSNM was done on favourable environments, mostly irrigated lowlands. Nonetheless, rice yields were also greater with SSNM than farmer practice under rainfed lowland conditions in the Philippines, India and Thailand (Banayo et al., 2018; Haefele and Konboon, 2009). However, the yield advantage was smaller compared to irrigated ecosystems. In some cases, both irrigated and rainfed, lower amounts of fertilizer are used with SSNM but still with greater yields than farmer practice. This can be attributed to the right time of application, particularly for N fertilizer, where N fertilizer timing coincides with crop demand, resulting in greater agronomic efficiency and hence greater utilization effeciciency of the applied N fertilizer.
Decision support tools
While the positive benefits of SSNM compared to the farmer practice, or local recommendation were demonstrated in different environments across different countries in Asia, the uptake of the SSNM recommendations remained low. Research partners linked with extension workers to extend the technology in different sites, but still the uptake remained low. This was largely because the SSNM approach is knowledge intensive and can not be easily used by extension to disseminate nutrient management recommendations to farmers. Using the principles of SSNM, IRRI developed and released an ICT decision support tool, Rice Crop Manager (RCM; http://cropmanager.irri.org) in the Philippines (Buresh et al., 2019), India (Sharma et al., 2019), Bangladesh, Indonesia and Vietnam to give field-specific fertilizer recommendations to individual smallholder farmers. It is a web-based decision support tool that that can be accessed using computers, tablets or smart phones connected to the internet. It is used to calculate field-specific nutrient management recommendations using information provided by a farmer through an interview. The farmer is asked 25-30 easy to answer questions about their field condition and crop management. At the end of the questionnaire Rice Crop Manager automatically calculates and gives easy to follow nutrient and crop management recommendations that can be printed on one page before the start of the season. RCM has been widely evaluated in the Philippines and has led to an increase in rice yield and income on average by 400 kg and $ 100 (PhP 6000) ha-1 season-1 over nine seasons on more than 1000 sites (Figure 1). In India the use of RCM resulted in positive rice and wheat yield gain with greater added net benefit when compared to the farmer practice (Table 1). RCM has potential to be released in other countries in the region including Cambodia, Myanmar, Sri Lanka, Thailand, with possibilities to expand to Africa. Its use can increase rice productivity to meet the challenges of rising demand for the commodity driven by population growth, while protecting the environment and contributing to attaining Sustainable Rice Platform (SRP) standards.
Table 1: Rice and wheat yield gain and added net benefit obtained with Rice Crop Manager (RCM) compared to the farmer practice in two states in India, Odisha and Bihar
Parameter
Unit
Effect of RCM (RCM-FP)
Odisha- Kharif Rice
Odisha-Rabi Rice
Bihar- Rice
Bihar- Wheat
Grain Yield
t ha-1
+0.8
*
+0.5
*
+0.7
*
+0.3
*
Net added Benefit
USD ha-1
155
*
104
*
147
*
63
*
* Significant at 0.05 level
Challenges
The increasing availability of ICT tools to provide accurate, timely and relevant crop management recommendations to farmers has been seen as an option for wider dissemination of information. While most farmers have access to mobile phones, only few have smart phones that can access the internet. Additionally, internet avilability and stability in rural areas, where many smallholder farmers are located, is generally poor and thus limiting the access to information. To improve access, RCM in the offline mode should be developed so that its use can be extended to areas with limited internet access.
As agriculture is increasingly being affected by climate change, changing and increasing insect pest and diseases, deteriorating natural resources and environmental pollution, there arises a need to equip these decision tools with updated and dynamic information which can cater to new generation agricultural problems. Fortunately there have been many new developments in soil and related subjects, which can increase the robustness of these tools and make them more dynamic. Improvement in precision of weather forecast systems, wherein one can have the information on the weather (rainfall, temperature, relative humidity, etc.) that is going to affect your crop in the coming days has potential to reduce the impact of adverse weather events. This will occur because the advance notice will allow decision makers the opportunity to implement plans to minimize the impact of adverse events and find opportunities within favorable events. Integration of weather information with other environmental information such as crop variety, soil type, soil maps, soil fertility, soil moisture and long-term drought conditions, can maximize the value of prediction information for decision-making process and will help farmers and agribusinesses utilize the information more effectively in decision-making.
The progress made in GIS and remote sensing has led to access to more accurate and timely information in the form of maps, statistics, and reports, crop production areas, crop health, crop losses due to natural calamities, and assessment and extent of pest damage. For example, IRRI developed Philippine Rice Information System (PRiSM) which uses satelite imagery to assess mid-season crop performance during the season and predict end of season yield. While RCM provides pre-season crop management recommendations using predictive and historical information, the actual performance in the field can be influenced by weather, pests, diseases and others. PRiSM can potentially be used to provide information that can be used to adjust RCM recommendations based on actual performance. A logical framework for integration and interface of the latest scientific developments in improved and resilient germplasm, improved and best crop and nutrient management, GIS and remote sensing, and weather forecasting can help to develop a robust and dynamic tools to guide the farmers with better and real time decision making. These tools have potential to revolutionize agriculture in the developing world.
REFERENCES
Banayo, N. P. M. C., Haefele, S., Desamero, N. V., and Kato, Y. (2018). On-farm assessment of site-specific nutrient management for rainfed lowland rice in the Philippines. Field Crops Research 220, 88-96.
Buresh, R. J., Laureles, E., Dela Torre, J. C., Samson, M., Guerra, M., Sinohin, P. J., and Castillo, R. (2019). Site-specific nutrient management for rice in the Philippines: Calculation of field-specific fertilizer requirements by Rice Crop Manager (under review). Field Crops Research.
Cassman, K. G., De Datta, S., Olk, D., Alcantara, J., Samson, M., Descalsota, J., and Dizon, M. (1995). Yield decline and the nitrogen economy of long-term experiments on continuous, irrigated rice systems in the tropics. Soil management: Experimental basis for sustainability and environmental quality, 181-222.
Dobermann, A. (2000). Future intensification of irrigated rice systems. In: Sheehy, J.E., Mitchell, P.L., Hardy, B. Eds.), Redesigning Rice Photosynthesis to Increase Yield. Interna- tional Rice Research Institute/Elsevier, Makati City, Philip- pines/Amsterdam, pp. 229-247.
Dobermann, A., Witt, C., and Dawe, D. (2004). Increasing the productivity of intensive rice systems through site-specific nutrient management. Science Publishers, Inc., Enfield, NH, USA and Int. Rice Res. Inst., Los Baños, Philippines.
Dobermann, A., Witt, C., Dawe, D., Abdulrachman, S., Gines, H., Nagarajan, R., Satawathananont, S., Son, T., Tan, P., and Wang, G. (2002). Site-specific nutrient management for intensive rice cropping systems in Asia. Field Crops Research 74, 37-66.
Gebbers, R., and Adamchuk, V. I. (2010). Precision agriculture and food security. Science 327, 828-831.
GRiSP, G. R. S. P. (2013). Rice almanac, 4th edition. Los Baños (Philippines). International Rice Research Institute. 283 p.
Haefele, S., and Konboon, Y. (2009). Nutrient management for rainfed lowland rice in northeast Thailand. Field Crops Research 114, 374-385.
Heffer, P. (2009). Assessment of fertilizer use by crop at the global level. International Fertilizer Industry Association, Paris, www. fertilizer. org/ifa/Home-Page/LIBRARY/Publication-database. html/Assessment-of-Fertilizer-Use-by-Crop-at-the-Global-Level-2006-07-2007-08. html2.
Khurana, H. S., Phillips, S. B., Alley, M. M., Dobermann, A., Sidhu, A. S., and Peng, S. (2008). Agronomic and economic evaluation of site-specific nutrient management for irrigated wheat in northwest India. Nutrient Cycling in Agroecosystems 82, 15-31.
Lu, C., and Tian, H. (2017). Global nitrogen and phosphorus fertilizer use for agriculture production in the past half century: shifted hot spots and nutrient imbalance. Ecology, Evolution and Organismal Biology Publications. 348.
National Research Council, N. (1997). Precision Agriculture in the 21st Century: Geospatial and Information Technologies in Crop Management. Washington, DC: The National Academies Press. https://doi.org/10.17226/5491.
Pampolino, M. F., Manguiat, I. J., Ramanathan, S., Gines, H., Tan, P., Chi, T., Rajendran, R., and Buresh, R. (2007). Environmental impact and economic benefits of site-specific nutrient management (SSNM) in irrigated rice systems. Agricultural Systems 93, 1-24.
Pingali, P. L. (2012). Green revolution: impacts, limits, and the path ahead. Proceedings of the National Academy of Sciences 109, 12302-12308.
Pretty, J., Toulmin, C., and Williams, S. (2011). Sustainable intensification in African agriculture. International journal of agricultural sustainability 9, 5-24.
Robert, P. C. (2002). Precision agriculture: a challenge for crop nutrition management. Plant and Soil 247, 143-149.
Seng, V., Ros, C., Bell, R., White, P., and Hin, S. (2001). Nutrient requirements of rainfed lowland rice in Cambodia. In: Fukai, S. and Basnayake, J., (eds.) Increased Lowland Rice Production in the Mekong Region; proceedings of an international workshop held in Vientiane, Laos, 30 Oct - 1 Nov. ACIAR, Canberra, pp. 169-178.
Sharma, S., Panneerselvam, P., Castillo, R., Manohar, S., Raj, R., Ravi, V., and Buresh, R. J. (2019). Web-based tool for calculating field-specific nutrient management for rice in India. Nutrient Cycling in Agroecosystems 113, 21-33.
Witt, C., Pasuquin, J., Pampolino, M., Buresh, R., and Dobermann, A. (2009). A manual for the development and participatory evaluation of sitespecific nutrient management for maize in tropical, favorable environments. International Plant Nutrition Institute, Penang. Dr. Timsina is shown visiting a maize field.