ABSTRACT
The reticuloruminal function is central to digestive efficiency in cattle. Monitoring the rumen condition in cattle is important because a dysfunctional rumen system may cause death. Subacute ruminal acidosis (SARA) is a typical disease in cattle, characterized by repeated periods of low ruminal pH. SARA is regarded as a trigger for rumen atony, rumenitis, and abomasal displacement, which may cause death. In previous studies, rumen conditions were evaluated using a wireless sensor with pH measurement capability. Such pH boluses provide direct information on the reticuloruminal function within the rumen. Extended periods of 3 hours or more with a ruminal pH 5.6 or lower indicates dysfunction and a likelihood of poor welfare. The primary advantage of an indwelling pH sensor is the ability to continuously measure ruminal or reticular pH. High-energy diets can have a negative impact on the function of the digestive system in cattle. Nevertheless, reinforced diets may be desirable for many reasons in modern farming, and the ability to continuously monitor their impact is therefore of interest. Here, we present an orally administrable indwelling wireless probe that can track pH and movement. The probe is designed to maintain position in the reticulum of cattle and to provide measurements without maintenance. The probe is cylindrical and consists of a pH sensor, temperature sensor, 3-axis accelerometer, microcontroller unit, transmitter, radiofrequency antenna, and battery. The probe housing is made from polytetrafluorethylene with a stainless-steel lid, and sealed with O-rings and silicone sealant. The probe is easily administered orally via catheter into the rumen. This system utilizes a collar repeater to relay transmissions to a receiver that stores data on a cloud-connected sever or a personal computer with special software. The communication distance from the collar repeater on a cow to the receiver was about 60 m. An in-situ test of the probe revealed that daily variations in pH, as well as changes induced by diet, were possible to detect. We also compared the acceleration data from our bolus probe with force transducer measurement of the ruminal motility, which shows that also can be detected by our probe. It appears as if this bolus-type wireless sensor is useful for detecting SARA and monitoring ruminal motility in cattle.
Keywords: Reticuloruminal function, bolus sensor, pH, motility, wireless
INTRODUCTION
Each animal in cattle farming represents a significant monetary investment for the farmer. To maximize returns on this investment, animals need to be kept healthy and in good condition while being as productive as possible with respect to, for example, milk yield. A large part of this involves ensuring proper nutrition. Natural food sources, such as grasses, are low in energy content. As a result, there are both economic and practical reasons for cattle farmers to include concentrates in the daily diet of their animals. However, the digestive system of graminivores such as cattle is quite sensitive and relies on specialized enzymes, symbiotic bacteria, and rumination movements for the difficult task of digesting cellulose from grasses. Large deviations in the conditions of the stomach can negatively impact the function of these enzymes and bacteria. Concentrates contain high levels of rapidly digestible carbohydrates and cause a dropin rumen pH when consumed in very large quantities. A reduction in rumen pH leads to decreased biochemical function and can eventually even lead to cessation of the rumen contractions necessary to control the flow of digesta. When this happens, the animal loses the ability to digest food and the condition can therefore prove fatal. An animal that exhibits prolonged periods of low rumen pH is said to suffer from subacute ruminal acidosis (SARA).
By continuously monitoring pH and rumen movements, it is possible to ensure that the diet is optimized for the maintenance of health even during periods of varying nutritional needs, such as in lactation. However, doing so can be very costly and resource-intensive, and such monitoring is thus currently not common practice. Under normal circumstances, relevant measurements cannot be taken from outside the animal without surgery. The solution is to use an indwelling probe. Such a probe must have no negative health impacts on the animal and must be able to perform the desired measurements and subsequent wireless data transfer to the outside for an extended period of time without maintenance. Both indwelling ruminal pH probes (Sato et al. 2012) and indwelling reticulorumen movement probes (Nogami et al. 2017) have been reported, and both types can provide viable data.
The frequency and amplitude of ruminal contractions in cattle are influenced by metabolic diseases such as ruminal acidosis and hypocalcaemia, as well as any of the many diseases that cause pain or fever (Andersen 2003, Daniel 1983, Leek 1983). In addition to anorexia, clinical signs of indigestion include reticuloruminal hypomotility (Falk et al. 2015). Reticuloruminal acidosis has shown to reduce the frequency of ruminal contraction, eventually resulting in ruminal stasis (Andersen 2003, Madan 2013). Ruminal contractions decrease in number and strength and reticuloruminal stasis develops, often within 15-30 min. Reticuloruminal stasis is of particular interest in relation to the pathogenesis of production diseases associated with gastrointestinal atony, such as displaced abomasum and ruminal tympany (Andersen 2003). The measurement of ruminal motility in its own right thus has value in the management of cattle to improve health, welfare and production performance.
Radiotelemetry has been used in research for many decades to monitor numerous physiological parameters such as respiratory rate, heart rate, and body temperature in animals (Brigh and Heal 1974). Recently, wireless sensors for intraruminal insertion through the oesophagus (boluses) have been developed as a non-invasive alternative to surgery. Variable measurement systems have become available for continuous monitoring of the ruminal and reticular pH or temperature in cattle (Alzahal et al. 2011, Falk et al. 2015, Mottram et al. 2008, Rose-Dye et al. 2011, Sato et al. 2012). These bolus sensors are useful for detecting SARA or fever in cattle. On the other hand, whether bolus sensors can carry out continuous long-term measurement of reticuloruminal motility in cattle remains unknown. Ruminal motility in cattle could be assessed physiologically using methods such as strain gauge force transducers or ultrasonography (Arai et al. 2019, Braun and Schweizer 2015). However, the force transducer method requires surgery in cattle, and continuous measurement for a long time using ultrasonography is difficult. More recently, bolus wireless sensors with accelerometers have been developed, and have been applied to assessing functions of the reticulorumen such as rumination or motion of the reticulorumen in cattle (Andersson et al. 2018, Hamilton et al. 2019, Nogami et al. 2017). However, whether a bolus wireless sensor with accelerometer can assess ruminal motility in cattle is not clear. If ruminal motility could be measured for a long time, this method may be useful for detecting dysfunctions of ruminal motility such as ruminal atony, ruminal tympany and anorexia. Here, an orally administered wireless reticulum probe is presented, with improvements in terms of reduced size and the capacity for both high-resolution pH measurements and movement measurements at a high sampling rate.
WIRELESS ACTIVITY AND PH PROBE FOR RETICULUM
Probe appearance and system
There are several constraints on the physical construction of the probe. The device must be made entirely of benign materials having no negative impact on the health of the animal. Given the intended oral administration, the shape and outer dimensions must be kept appropriate. The probe housing is made from biocompatible polytetrafluorethylene (PTFE) with a stainless-steel lid and sealed with O-rings and silicone sealant. The probe has a diameter of 30 mm, length of 100 mm, and weighs 124 g. The whole pH electrode assembly is encased in the PTFE housing with ruminal liquid access through 5-mm-diameter holes placed in a pattern matching the shape of the pH sensing assembly, so as to provide maximum protection for the glass sensing electrode. Figure 1 shows disassembled and assembled views of the probe.
The probe contains a commercially available pH and reference electrode assembly controlled through a custom-built interface. This assembly has a medically safe body and contains a conventional pH-sensing glass electrode and a double-junction Ag/AgCl reference electrode. Movement is monitored using an accelerometer (ADXL362; Analog Devices, Chelmsford, MA, USA) integrated into a custom-built microcontroller unit (MCU) (C8051F930; Silicon Labs, TX, USA) and a transmitter circuit (Si4461-B1; Silicon Labs). Power is provided by a heavy-duty 3-V lithium battery, and all active components are designed for sub-3-V operation to ensure functionality is maintained when battery potential decreases during discharge.
This system shows great flexibility in measurements. Data can be independently measured and transmitted from any of the sensors contained in the probe. Although sampling rates and measurement resolutions should be minimized to conserve power for long-term operations, they were kept high during this short-term trial to allow the prototype probe to capture detailed data that could be used to develop optimized measurement schemes for future iterations. Acceleration is probed at 1-s intervals and in a ±2-G range with 10-bit resolution, while pH is sampled once per minute in a pH range of 2–10 and a resolution of 0.03 pH units. All measurements are transmitted to the receiver in real time.
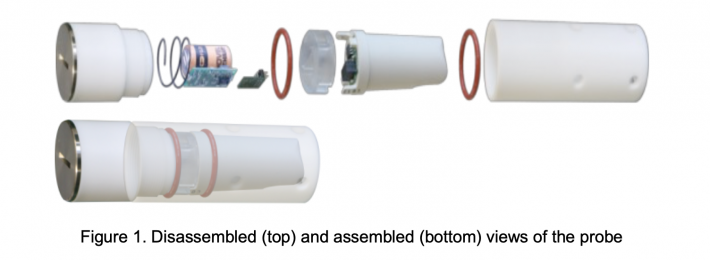
Data transfer
Transmissions are in the 429-MHz band. This frequency band does not require any license, but the maximum effective isotropic radiated power is restricted to 12.14 dBm. Since the radiation power of the sensor is attenuated considerably by body tissue and rumen fluid when the probe is in the intended location, the maximum allowed transmission power is insufficient to maintain a viable reception range for a regular farm environment. To overcome this, the system utilizes a collar repeater to relay transmissions to a receiver that stores the data on a cloud-connected server accessible for manual or automated evaluation on any viable internet-enabled device. Figure 2 shows an overview of the system in operation.
A typical measurement and transmission cycle consumes less than 350 nAh, almost all of which is attributed to transmission. Given the 1000-mAh capacity of the battery and the negligible standby current (<1 mA), this means that the probe in the configuration used here has a calculated lifetime of slightly over 1 month. Real tests confirm that the lifetime is well over 1 month. Several methods can be used to increase the lifetime of the probe. Ways to achieve this include reducing the sampling rate and number of transmission events, as well as reducing measurement resolution. Another alternative is to use more elaborate measurement schemes with relatively high sampling rates, but only intermittent measurements. Moreover, the probe can easily be configured for any desired measurement protocol and the target lifetime is easily achieved.
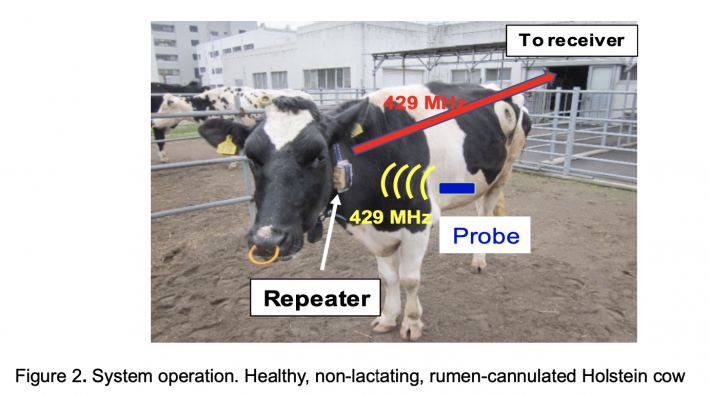
Probe insertion and location
The intended location of the probe is the reticulum, a small compartment at the front of the rumen. For these trials, the probe was able to be inserted and its location manually verified through the fistula. During the trial, the probe was extracted once per week to check the drift of the pH sensor, and insertion was thus performed 4 times. Depending on the contents of the reticulorumen, primarily the amount of dry matter, a significant amount of time may be needed for the probe to sink to the bottom where it needs to be in order to deliver reliable measurements. When inserted into the rumen, the probe will eventually (within a few days) spontaneously transfer from the rumen to the reticulum, where it remains indefinitely.
Although it is not possible to say for certain on the basis of this very limited trial, the location of the probe may be able to be determined from the measurement data alone. Mean pH is lower in the rumen than in the reticulum. Data in the literature suggest a difference of about 0.2 pH units in healthy cows (Falk et al. 2015, Sato et al. 2012), consistent with the data obtained during this trial by comparing data collected after the probe was inserted directly into the reticulum and after insertion into the rumen before spontaneous transfer to the reticulum. A discernible difference in movement patterns is also apparent between the rumen and the reticulum. The latter exhibits more feature-rich movements, where each movement generally displays three local maxima, while the former displays smoother movements with fewer maxima and a slightly different rhythm.
Since the accelerometer is subject to Earth gravity, orientation can be monitored. Once in the intended location in the reticulum, accelerometer data reveal that most of the time, the probe is oriented at a roughly 10° angle relative to the horizontal position with the pH sensor slightly elevated compared with the stainless-steel lid. The probe sometimes shifts horizontally or vertically, but almost always with the pH sensor end pointing up. This is consistent with the centre of gravity, which is slightly towards the stainless-steel lid. Although not experimentally verified, this might be advantageous, since the bottom of the reticulum contains a significant amount of debris such as small stones and keeping the pH sensor away from this sediment might be beneficial to reduce the risk of physical damage to the delicate sensing electrode.
Reticular pH
The cow on which this study was performed was fed regularly at 09:00 and 15:00, and the reticulum pH reflects this in systematic variations during the course of the day. Figure 3 shows that concomitant with feeding, pH drops by roughly 0.15 units when under regular diet. Recovery is slow enough so that equilibrium is never reached and pH thus varies constantly throughout the day. To test the capability of the probe to detect SARA, the cow was fed an experimental high-energy diet for 1 day, which increased the feeding-related pH drop to about 0.4 pH units per meal (Fig. 3).
Fast changes are clearly able to be detected, and short-term (1 month in this trial) monitoring for acidosis appears possible using this probe. However, slow, long-term changes in pH due to less extreme deliberate dietary changes might be more problematic because of drift of the pH sensor, and whether this probe can perform the intended function over the long term using this pH sensor is presently unclear.
Although rumen pH varies throughout the day and conceivably also between populations and individuals, attempts have been made to define thresholds for diagnosing SARA. One example of such a threshold is when rumen pH is more than 0.9 pH units below normal for an extended period of time (Duffield et al. 2004). The maximum decrease in pH due to the experimental diet in this trial was less than that (Fig. 3), but this is consistent with other studies that suggest that the SARA-related drop in pH is smaller in the reticulum than in the rumen (Sato et al. 2012). On the basis of the relative stability and consistency of reticulum pH when on a regular diet and the response to the experimental diet observed in this trial, such a threshold should be able to be defined for the reticulum and this probe.
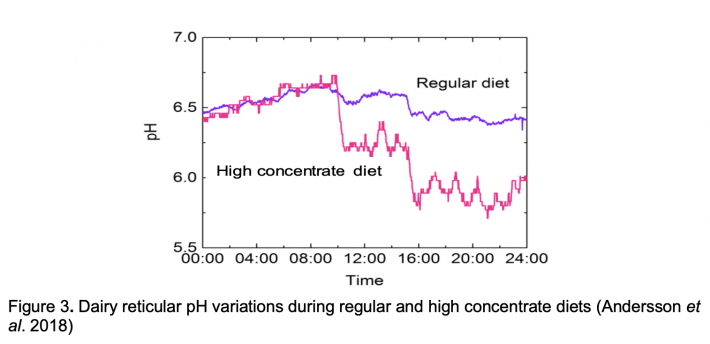
EVALUATION OF RUMINAL MOTILITY USING A WIRELESS SENSOR
Development of the small probe
The probe is cylindrical and consists of a 3-axis accelerometer (ADXL362; Analog Devices, Chelmsford, MA, USA), temperature sensor, MCU (C8051F930; Silicon Labs), transmitter (Si4461-B1; Silicon Labs), RF antenna, and battery. The probe housing is made from PTFE with glue and PTFE sealing tape. Probe characteristics are: diameter, 22 mm; length, 76 mm; and weight, 55 g (density, 1.9 g/cm3). The probe is easily administered orally via a catheter into the rumen. Power is provided by a heavy-duty 3-V lithium battery. The acceleration is probed at 1-s intervals and in a ±2-G range with 10-bit resolution. Transmissions are in the 429-MHz band. This frequency band does not require any license in Japan, but the maximum effective radiated power is restricted to 12.14 dBm. The battery had a mean life of 1 month when measurements of 3-axis acceleration were transmitted continuously every 1 s. This system utilizes a collar repeater to relay the transmissions to receiver that stores data on a cloud-connected server or a personal computer with special software. Communication distance from the collar repeater on the cow to the receiver was about 60 m.
Measurement acceleration data and ruminal motility
Acceleration data from the bolus sensor were transmitted to the receiver in real time. Three-axis acceleration data were recorded to a personal computer with special software connected the receiver. Sampling rates for acceleration was 1 Hz. In terms of three-axis acceleration, the x and z axes indicate the radial acceleration of the probe, and the y axis indicates vertical acceleration. Acceleration (mG) and peak number of accelerations (times/min) from the bolus sensor were calculated as mean values for 10 min. The baseline of the y-axis was kept level, but baselines of the x- and z-axes followed a large curve over the day. The probe was in an almost horizontal position with respect to the longitudinal axis. The basic movement of acceleration of the y-axis showed a very distinct vertical pattern occurring roughly once per minute, with a duration of approximately 8 s, and displaying two local maxima at around 500 mG. On the other hand, acceleration of the x- and z-axes displayed indistinct vertical patterns.
Ruminal motility in cattle was measured using strain gauge force transducers (Arai et al. 2019). Briefly, the force transducer used in this study measured 18 × 35 mm and contained a strain gauge. The force transducer was covered with silicone. Four cattle were sedated intramuscularly with xylazine hydrochloride (0.05 mg/kg). After 10 min, local anaesthesia was applied to the left paralumbar fossa by intramuscular infusion of procaine hydrochloride (80~100 ml/body). The force transducer was sutured to the serosa of the dorsal sac of the rumen, then the lead wires were exteriorized through the abdominal wall. These the wires from the force transducer were then subcutaneously tunnelled and pulled out through the skin. The cattle were injected intramuscularly with antibiotics (10 mg/kg, ampicillin sodium) after the surgery. Ruminal motility was recorded on a polygraph (MP100A; BIOPAC Systems Inc., Santa Barbara, CA, USA) by connecting the lead wires of the transducers to the connecting cables from the amplifiers (DA100; BIOPAC Systems Inc.), then digitised by analogue-to-digital converter, and continuously recorded on a computer for analysis.
Relationship between ruminal motility and acceleration data
We examined the correlation between ruminal motility by force transducer and acceleration by bolus sensor. The bolus sensor was orally administered using a custom-made gastric tube without surgery. The location of the bolus sensor in the reticulum was confirmed by the movement pattern of acceleration (Andersson et al. 2018). A force transducer was sutured to the dorsal sac of the rumen in surgery. Measurements using the force transducer and bolus sensor in the experiment were carried out with cattle 7 days after surgery. Ruminal motility by force transducer and acceleration by bolus sensor were recorded continuously and simultaneously throughout the trial.
Figure 4 shows measurement results of ruminal motility by force transducer and acceleration data by bolus sensor. The waveform of ruminal motility by force transducer and that of acceleration by bolus sensor appeared to largely correspond. In addition, a significant positive correlation was observed between amplitude of ruminal contraction by force transducer and acceleration by bolus sensor. A significant positive correlation was observed between frequency of ruminal contraction by force transducer and peak number of accelerations by bolus sensor. Contraction of the dorsal sac of the rumen and acceleration signals in the reticulum thus occurred at practically the same time. This phenomenon was considered to represent the flow of ruminal contents occurring with compression of the dorsal sac of the rumen pushing and moving the bolus sensor within the reticulum. Braun et al. reported the duration of contraction of the dorsal sac of the rumen as 8.2 ± 1.04 s (Braun and Schweizer 2015). In the present study, duration of acceleration of the bolus sensor was about 8 s. Movement of the dorsal sac of the rumen and acceleration of digesta in the reticulum thus appeared largely correspondent.
The general pattern of reticuloruminal motility consists of regular contraction sequences (Constable et al. 1990, Steller and Stevens 1966) that successively engage the various parts of the reticulum and rumen. There are primary and secondary contraction cycles, with the former serving primarily to mix ingesta, support optimal microbial fermentation and transport ingesta to the omasum, whereas the latter are involved in eructation of gas (Constable et al. 1990). The primary cycles starts with biphasic reticular contraction, followed immediately by contraction of the ruminal atrium. The dorsal sac of the rumen, the caudodorsal blind sac and the left longitudinal groove contract at practically the same time, after which contraction of the caudoventral blind sac and ventral sac of the rumen occurs. The secondary contraction cycle does not involve the reticulum and ruminal atrium and consists of contraction of the dorsal sac and then the ventral sac of the rumen (Braun and Schweizer 2015, Constable et al. 1990). In the present study, acceleration of the bolus sensor generally displayed two local maxima. Since the bolus sensor was located in the reticulum, these local maxima may reflect biphasic reticular contraction. The major waveform of acceleration by bolus sensor probably indicates the flow of ruminal contents occurring by compression of the dorsal sac of the rumen, because a significant positive correlation was observed between acceleration and ruminal motility.
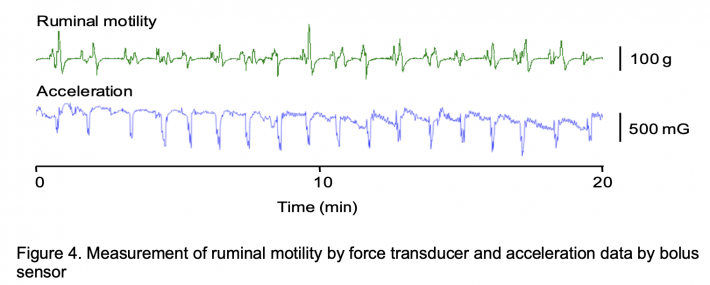
Detection of ruminal atony by bolus sensor
We examined the effect of ruminal motility and acceleration after intramuscular administration of xylazine hydrochloride (0.05 mg/kg; Bayer, Leverkusen, Germany) in cattle as a single dose at 12:00. Measurement data of ruminal motility and acceleration were collected for 120 min before and after administration of xylazine hydrochloride.
Ruminal atony occurred at about 10 min after administration of xylazine hydrochloride. This ruminal atony was detected almost synchronously by bolus sensor and force transducer. As an α2-adrenoreceptor agonist, xylazine is often used in cattle as a sedative. The pharmacological effects of xylazine include excessive depression of the central nervous system (CNS), ruminal atony and bradycardia (Thompson et al. 1991). In the case of disorders of ruminal motility, general anaesthesia and diseases at any sites that produce pain or fever can inhibit the hindbrain reflex centres responsible for evoking primary and secondary cycle contractions of the reticulorumen (Leek 1983). Clinical cases of ruminal stasis should be interpreted as being caused by either depression of the gastric centres, or a lack of excitatory reflex inputs, or an increase in inhibitory reflex inputs, or blockade of the motor pathways as in hypocalcaemic animals, but probably not in the case of vagus indigestion. Besides these specific reflex inputs, the gastric centres are influenced by nervous activity in other parts of the CNS (Leek 1983).
In addition, ruminal tympany (bloat) occurred after ruminal atony. This sign is of particular significance in fattening beef cattle, but may also be a problem in dairy herds on high concentrate rations (Enemark 2008). In healthy cattle, gaseous distension of the reticulorumen reflex elicits an increase in the force and frequency of secondary cycles, evokes opening of the cardia and initiates other respiratory, oesophageal, pharyngeal and buccal actions in the eructation sequence. Normally the cardiac region is cleared when the dorsal ruminal sac contractions of the secondary cycles force the gas layer forward, thereby depressing the fluid level below the level of cardia. In the case of systemic disease where ruminal hypomotility or stasis are secondary features, a persistent, mild bloat may be evident. This is because although the fermentation rate is probably low, weak ruminal motility may be inadequate to move the gas layer and clear the cardia (Leek 1983). The bolus sensor could evaluate ruminal motility such as frequency, amplitude and duration of hypomotility. Evaluation of ruminal motility using the bolus sensor may be useful as a non-invasive method of investigating reticuloruminal stasis in cows with ruminal tympany, acidosis, endotoxaemia, fever and hypocalcaemia (Andersen 2003, Daniel 1983, Leek 1983).
CONCLUSION
Although the probe can perform its intended tasks well in the short term, as demonstrated by this 1-month trial, two primary concerns need to be addressed before this system can be made commercially available. First, the size of the probe should be further reduced. The probe can be administered orally in the current form, but is still large and may prove uncomfortable for the animal. The size-limiting part is the pH sensing assembly and a smaller alternative thus needs to be found or developed. Second, again related to the pH sensor, are concerns regarding the long-term stability of pH measurements. As it stands, the probe can likely detect rapid changes in pH for the duration of its lifetime, but detection of slow changes such as those that would presumably occur if the diet is optimized over the course of several weeks might not be possible. Increasing the stability of the pH sensor is therefore desirable. The bolus sensor could be orally administered without surgery, and was continuously retained in the reticulum. A significant positive correlation was observed between ruminal contraction by force transducer and acceleration by bolus sensor. Contraction of the dorsal sac of the rumen and acceleration signals in the reticulum occurred at practically the same time. The bolus sensor could also detect ruminal atony after xylazine administration. We therefore suggest that the bolus-type wireless sensor may be useful for measuring ruminal motility and detecting dysfunction of the rumen such as ruminal atony and ruminal tympany.
ACKNOWLEDGMENT
This research was supported by a grant (The Research Project for The Future Agricultural Production Utilizing Artificial Intelligence; grant no. ai01) from the Project of the Bio-oriented Technology Research Advancement Institution, NARO.
REFERENCES
Alzahal, O., Alzahal, H., Steele M.A., Van Schaik, M., Kyriazakis, I., Duffield, T.F. and McBride, B.W. 2011. The use of a radiotelemetric ruminal bolus to detect body temperature changes in lactating dairy cattle. J Dairy Sci. 94: 3568-3574.
Andersen, P.H. 2003. Bovine endotoxicosis-some aspects of relevance to production diseases. A review. Acta.Vet. Scand. 98: 141-155.
Andersson, L.M., Arai, S. and Okada, H. 2018. Orally administrable wireless activity and pH probe for cattle reticulum. Sens. Mather. 30: 3029-3038.
Arai, S., Haritani, M., Sawada, H. and Kimura, K. 2019. Effect of mosapride on ruminal motility in cattle. J. Vet. Med. Sci. 81: 1017-1020.
Bewley, J. M., Grott, M. W., Einstein, M. E. and Schutz, M. M. 2008. Impact of intake water temperatures on reticular temperatures of lactating dairy cows. J. Dairy Sci. 91: 3880-3887.
Bligh, J. and Heal, J.W. 1974. Use of radio-telemetry in study of animal physiology. Proc. Nutr. Soc. 33: 173-181.
Braun, U. and Schweizer, A. 2015. Ultrasonographic assessment of reticuloruminal motility in 45 cows. Schweiz. Arch. Tierheilkd. 157: 87-95.
Constable, P. D., Hoffsis, G. F. and Rings, D. M. 1990. The reticulorumen: Normal and abnormal motor function. Part I. Primary contraction cycle. Comp. Cont. Educ. Pract. Vet. 12: 1008-1014.
Constable, P. D., Hoffsis, G. F. and Rings, D. M. 1990. The reticulorumen: Normal and abnormal motor function. Part II. Secondary contraction cycles, rumination, and esophageal groove closure. Comp. Cont. Educ. Pract. Vet. 12: 1169-1174.
Daniel, R. C. W. 1983. Motility of the rumen and abomasum during hypocalcaemia. Can. J. Comp. Med. 47: 276-280.
DePeters, E.J. and George, L.W. 2014. Rumen transfaunation. Immunol. Lett. 162: 69-76.
Duffield, T., Plaizier, J. C., Fairfield, A., Bagg, R., Vessie, G., Dick, P., Wilson, J., Aramini, J. and McBride, B. 2004. Comparison of techniques for measurement of rumen pH in lactating dairy cows. J. Dairy Sci. 87: 59-66.
Egert, A. M., Klots, J. L., McLeod, K. R. and Harmon, D. L. 2014. Development of a methodology to measure the effect of ergot alkaloids on forestomach motility using real-time wireless telemetry. Front Chemi. 2(90): 1-8.
Enemark, J. M. D. 2008. The monitoring, prevention and treatment of sub-acute ruminal acidosis (SARA): A review. Vet. J. 176: 32-43.
Falk, M., Munger, A. and Dohme-Meier, F. 2015. Technical note: A comparison of reticular and ruminal pH monitored continuously with 2 measurement systems at different weeks of early lactation. J. Dairy Sci. 99: 1951-1955.
Hamilton, A.W., Davison, C., Tachtazis, C., Andonovic, I., Michie, C., Ferguson, H.J. Somerville, L. and Jonsson N.N. 2019. Identification of the rumination in cattle using support vector machines with motion-sensitive bolus sensors. Sensors 19, 1165.
Klevenhusen, F., Pourazad, P., Wetzels, S. U., Qumar, M., Khol-Parisini, A. and Zebeli, Q. 2014. Technical note: Evaluation of a real-time wireless pH measurement system relative to intraruminal differences of digesta in dairy cattle. J. Anim. Sci. 92: 5635-5639.
Leek, B.F. 1983. Clinical diseases of the rumen: a physiologist’s view. Vet. Rec. 113: 10-14.
Madan, J., Gupta, M., Rose, M.K., Sindhu, S. and Kar, D. 2013. Metabolic alterations in buffaloes suffering from digestive disorders. Haryana Vet. 52: 71-72.
Mottram, T., Lowe, J., McGowan, M. and Phillips, N. 2008. Technical note: A wireless telemetric method of monitoring clinical acidosis in dairy cows. Comput. Electron. Agric. 64: 45-48.
Nogami, H., Arai, S., Okada, H., Zhan, L. and Itoh, T. 2017. Minimized bolus-type wireless sensor node with a built-in three-axis acceleration meter for monitoring a cow’s rumen condition. Sensors 17, 687.
Rose-Dye, T.K., Burciaga-Robles, L.O., Krehbiel, C.R., Step, D.L., Fulton, R.W., Confer, A.W. and Richards, C.J. 2011. Rumen temperature change monitored with remote rumen temperature boluses after challenges with bovine viral diarrhea virus and Mannheimia haemolytica. J. Anim. Sci. 89: 1193-1200.
Sato, S., Ikeda, A., Tsuchiya, Y., Ikuta, K., Murayama, I., Kanehira, M., Okada, K. and Mizuguchi, H. 2012. Diagnosis of subacute ruminal acidosis (SARA) by continuous reticular pH measurements in cows. Vet. Res. Commun. 36: 201-205.
Sato, S., Kimura, A., Anan, T., Yamagishi, N., Okada, K., Mizuguchi, H. and Ito, K. 2012. A radio transmission pH measurement system for continuous evaluation of fluid pH in the rumen of cows. Vet. Res. Commun. 36: 85-89.
Stellers, A. F. and Stevens, C. E. 1966. Motor functions of the ruminant forestomach. Physiol. Rev. 46: 634-661.
Thompson, J. R., Kersting, K. W. and Hsu, W. H. 1991. Antagonistic effect of atipamezole on xylazine-induced sedation, bradycardia, and ruminal stony in calves. Am. J. Vet. Res. 52: 1265-1268.
Date submitted: October 24, 2019
Reviewed, edited and uploaded: November 25, 2019 |
Measurement of Reticuloruminal Function in Cattle Using a Bolus-Type Wireless Sensor
ABSTRACT
The reticuloruminal function is central to digestive efficiency in cattle. Monitoring the rumen condition in cattle is important because a dysfunctional rumen system may cause death. Subacute ruminal acidosis (SARA) is a typical disease in cattle, characterized by repeated periods of low ruminal pH. SARA is regarded as a trigger for rumen atony, rumenitis, and abomasal displacement, which may cause death. In previous studies, rumen conditions were evaluated using a wireless sensor with pH measurement capability. Such pH boluses provide direct information on the reticuloruminal function within the rumen. Extended periods of 3 hours or more with a ruminal pH 5.6 or lower indicates dysfunction and a likelihood of poor welfare. The primary advantage of an indwelling pH sensor is the ability to continuously measure ruminal or reticular pH. High-energy diets can have a negative impact on the function of the digestive system in cattle. Nevertheless, reinforced diets may be desirable for many reasons in modern farming, and the ability to continuously monitor their impact is therefore of interest. Here, we present an orally administrable indwelling wireless probe that can track pH and movement. The probe is designed to maintain position in the reticulum of cattle and to provide measurements without maintenance. The probe is cylindrical and consists of a pH sensor, temperature sensor, 3-axis accelerometer, microcontroller unit, transmitter, radiofrequency antenna, and battery. The probe housing is made from polytetrafluorethylene with a stainless-steel lid, and sealed with O-rings and silicone sealant. The probe is easily administered orally via catheter into the rumen. This system utilizes a collar repeater to relay transmissions to a receiver that stores data on a cloud-connected sever or a personal computer with special software. The communication distance from the collar repeater on a cow to the receiver was about 60 m. An in-situ test of the probe revealed that daily variations in pH, as well as changes induced by diet, were possible to detect. We also compared the acceleration data from our bolus probe with force transducer measurement of the ruminal motility, which shows that also can be detected by our probe. It appears as if this bolus-type wireless sensor is useful for detecting SARA and monitoring ruminal motility in cattle.
Keywords: Reticuloruminal function, bolus sensor, pH, motility, wireless
INTRODUCTION
Each animal in cattle farming represents a significant monetary investment for the farmer. To maximize returns on this investment, animals need to be kept healthy and in good condition while being as productive as possible with respect to, for example, milk yield. A large part of this involves ensuring proper nutrition. Natural food sources, such as grasses, are low in energy content. As a result, there are both economic and practical reasons for cattle farmers to include concentrates in the daily diet of their animals. However, the digestive system of graminivores such as cattle is quite sensitive and relies on specialized enzymes, symbiotic bacteria, and rumination movements for the difficult task of digesting cellulose from grasses. Large deviations in the conditions of the stomach can negatively impact the function of these enzymes and bacteria. Concentrates contain high levels of rapidly digestible carbohydrates and cause a dropin rumen pH when consumed in very large quantities. A reduction in rumen pH leads to decreased biochemical function and can eventually even lead to cessation of the rumen contractions necessary to control the flow of digesta. When this happens, the animal loses the ability to digest food and the condition can therefore prove fatal. An animal that exhibits prolonged periods of low rumen pH is said to suffer from subacute ruminal acidosis (SARA).
By continuously monitoring pH and rumen movements, it is possible to ensure that the diet is optimized for the maintenance of health even during periods of varying nutritional needs, such as in lactation. However, doing so can be very costly and resource-intensive, and such monitoring is thus currently not common practice. Under normal circumstances, relevant measurements cannot be taken from outside the animal without surgery. The solution is to use an indwelling probe. Such a probe must have no negative health impacts on the animal and must be able to perform the desired measurements and subsequent wireless data transfer to the outside for an extended period of time without maintenance. Both indwelling ruminal pH probes (Sato et al. 2012) and indwelling reticulorumen movement probes (Nogami et al. 2017) have been reported, and both types can provide viable data.
The frequency and amplitude of ruminal contractions in cattle are influenced by metabolic diseases such as ruminal acidosis and hypocalcaemia, as well as any of the many diseases that cause pain or fever (Andersen 2003, Daniel 1983, Leek 1983). In addition to anorexia, clinical signs of indigestion include reticuloruminal hypomotility (Falk et al. 2015). Reticuloruminal acidosis has shown to reduce the frequency of ruminal contraction, eventually resulting in ruminal stasis (Andersen 2003, Madan 2013). Ruminal contractions decrease in number and strength and reticuloruminal stasis develops, often within 15-30 min. Reticuloruminal stasis is of particular interest in relation to the pathogenesis of production diseases associated with gastrointestinal atony, such as displaced abomasum and ruminal tympany (Andersen 2003). The measurement of ruminal motility in its own right thus has value in the management of cattle to improve health, welfare and production performance.
Radiotelemetry has been used in research for many decades to monitor numerous physiological parameters such as respiratory rate, heart rate, and body temperature in animals (Brigh and Heal 1974). Recently, wireless sensors for intraruminal insertion through the oesophagus (boluses) have been developed as a non-invasive alternative to surgery. Variable measurement systems have become available for continuous monitoring of the ruminal and reticular pH or temperature in cattle (Alzahal et al. 2011, Falk et al. 2015, Mottram et al. 2008, Rose-Dye et al. 2011, Sato et al. 2012). These bolus sensors are useful for detecting SARA or fever in cattle. On the other hand, whether bolus sensors can carry out continuous long-term measurement of reticuloruminal motility in cattle remains unknown. Ruminal motility in cattle could be assessed physiologically using methods such as strain gauge force transducers or ultrasonography (Arai et al. 2019, Braun and Schweizer 2015). However, the force transducer method requires surgery in cattle, and continuous measurement for a long time using ultrasonography is difficult. More recently, bolus wireless sensors with accelerometers have been developed, and have been applied to assessing functions of the reticulorumen such as rumination or motion of the reticulorumen in cattle (Andersson et al. 2018, Hamilton et al. 2019, Nogami et al. 2017). However, whether a bolus wireless sensor with accelerometer can assess ruminal motility in cattle is not clear. If ruminal motility could be measured for a long time, this method may be useful for detecting dysfunctions of ruminal motility such as ruminal atony, ruminal tympany and anorexia. Here, an orally administered wireless reticulum probe is presented, with improvements in terms of reduced size and the capacity for both high-resolution pH measurements and movement measurements at a high sampling rate.
WIRELESS ACTIVITY AND PH PROBE FOR RETICULUM
Probe appearance and system
There are several constraints on the physical construction of the probe. The device must be made entirely of benign materials having no negative impact on the health of the animal. Given the intended oral administration, the shape and outer dimensions must be kept appropriate. The probe housing is made from biocompatible polytetrafluorethylene (PTFE) with a stainless-steel lid and sealed with O-rings and silicone sealant. The probe has a diameter of 30 mm, length of 100 mm, and weighs 124 g. The whole pH electrode assembly is encased in the PTFE housing with ruminal liquid access through 5-mm-diameter holes placed in a pattern matching the shape of the pH sensing assembly, so as to provide maximum protection for the glass sensing electrode. Figure 1 shows disassembled and assembled views of the probe.
The probe contains a commercially available pH and reference electrode assembly controlled through a custom-built interface. This assembly has a medically safe body and contains a conventional pH-sensing glass electrode and a double-junction Ag/AgCl reference electrode. Movement is monitored using an accelerometer (ADXL362; Analog Devices, Chelmsford, MA, USA) integrated into a custom-built microcontroller unit (MCU) (C8051F930; Silicon Labs, TX, USA) and a transmitter circuit (Si4461-B1; Silicon Labs). Power is provided by a heavy-duty 3-V lithium battery, and all active components are designed for sub-3-V operation to ensure functionality is maintained when battery potential decreases during discharge.
This system shows great flexibility in measurements. Data can be independently measured and transmitted from any of the sensors contained in the probe. Although sampling rates and measurement resolutions should be minimized to conserve power for long-term operations, they were kept high during this short-term trial to allow the prototype probe to capture detailed data that could be used to develop optimized measurement schemes for future iterations. Acceleration is probed at 1-s intervals and in a ±2-G range with 10-bit resolution, while pH is sampled once per minute in a pH range of 2–10 and a resolution of 0.03 pH units. All measurements are transmitted to the receiver in real time.
Data transfer
Transmissions are in the 429-MHz band. This frequency band does not require any license, but the maximum effective isotropic radiated power is restricted to 12.14 dBm. Since the radiation power of the sensor is attenuated considerably by body tissue and rumen fluid when the probe is in the intended location, the maximum allowed transmission power is insufficient to maintain a viable reception range for a regular farm environment. To overcome this, the system utilizes a collar repeater to relay transmissions to a receiver that stores the data on a cloud-connected server accessible for manual or automated evaluation on any viable internet-enabled device. Figure 2 shows an overview of the system in operation.
A typical measurement and transmission cycle consumes less than 350 nAh, almost all of which is attributed to transmission. Given the 1000-mAh capacity of the battery and the negligible standby current (<1 mA), this means that the probe in the configuration used here has a calculated lifetime of slightly over 1 month. Real tests confirm that the lifetime is well over 1 month. Several methods can be used to increase the lifetime of the probe. Ways to achieve this include reducing the sampling rate and number of transmission events, as well as reducing measurement resolution. Another alternative is to use more elaborate measurement schemes with relatively high sampling rates, but only intermittent measurements. Moreover, the probe can easily be configured for any desired measurement protocol and the target lifetime is easily achieved.
Probe insertion and location
The intended location of the probe is the reticulum, a small compartment at the front of the rumen. For these trials, the probe was able to be inserted and its location manually verified through the fistula. During the trial, the probe was extracted once per week to check the drift of the pH sensor, and insertion was thus performed 4 times. Depending on the contents of the reticulorumen, primarily the amount of dry matter, a significant amount of time may be needed for the probe to sink to the bottom where it needs to be in order to deliver reliable measurements. When inserted into the rumen, the probe will eventually (within a few days) spontaneously transfer from the rumen to the reticulum, where it remains indefinitely.
Although it is not possible to say for certain on the basis of this very limited trial, the location of the probe may be able to be determined from the measurement data alone. Mean pH is lower in the rumen than in the reticulum. Data in the literature suggest a difference of about 0.2 pH units in healthy cows (Falk et al. 2015, Sato et al. 2012), consistent with the data obtained during this trial by comparing data collected after the probe was inserted directly into the reticulum and after insertion into the rumen before spontaneous transfer to the reticulum. A discernible difference in movement patterns is also apparent between the rumen and the reticulum. The latter exhibits more feature-rich movements, where each movement generally displays three local maxima, while the former displays smoother movements with fewer maxima and a slightly different rhythm.
Since the accelerometer is subject to Earth gravity, orientation can be monitored. Once in the intended location in the reticulum, accelerometer data reveal that most of the time, the probe is oriented at a roughly 10° angle relative to the horizontal position with the pH sensor slightly elevated compared with the stainless-steel lid. The probe sometimes shifts horizontally or vertically, but almost always with the pH sensor end pointing up. This is consistent with the centre of gravity, which is slightly towards the stainless-steel lid. Although not experimentally verified, this might be advantageous, since the bottom of the reticulum contains a significant amount of debris such as small stones and keeping the pH sensor away from this sediment might be beneficial to reduce the risk of physical damage to the delicate sensing electrode.
Reticular pH
The cow on which this study was performed was fed regularly at 09:00 and 15:00, and the reticulum pH reflects this in systematic variations during the course of the day. Figure 3 shows that concomitant with feeding, pH drops by roughly 0.15 units when under regular diet. Recovery is slow enough so that equilibrium is never reached and pH thus varies constantly throughout the day. To test the capability of the probe to detect SARA, the cow was fed an experimental high-energy diet for 1 day, which increased the feeding-related pH drop to about 0.4 pH units per meal (Fig. 3).
Fast changes are clearly able to be detected, and short-term (1 month in this trial) monitoring for acidosis appears possible using this probe. However, slow, long-term changes in pH due to less extreme deliberate dietary changes might be more problematic because of drift of the pH sensor, and whether this probe can perform the intended function over the long term using this pH sensor is presently unclear.
Although rumen pH varies throughout the day and conceivably also between populations and individuals, attempts have been made to define thresholds for diagnosing SARA. One example of such a threshold is when rumen pH is more than 0.9 pH units below normal for an extended period of time (Duffield et al. 2004). The maximum decrease in pH due to the experimental diet in this trial was less than that (Fig. 3), but this is consistent with other studies that suggest that the SARA-related drop in pH is smaller in the reticulum than in the rumen (Sato et al. 2012). On the basis of the relative stability and consistency of reticulum pH when on a regular diet and the response to the experimental diet observed in this trial, such a threshold should be able to be defined for the reticulum and this probe.
EVALUATION OF RUMINAL MOTILITY USING A WIRELESS SENSOR
Development of the small probe
The probe is cylindrical and consists of a 3-axis accelerometer (ADXL362; Analog Devices, Chelmsford, MA, USA), temperature sensor, MCU (C8051F930; Silicon Labs), transmitter (Si4461-B1; Silicon Labs), RF antenna, and battery. The probe housing is made from PTFE with glue and PTFE sealing tape. Probe characteristics are: diameter, 22 mm; length, 76 mm; and weight, 55 g (density, 1.9 g/cm3). The probe is easily administered orally via a catheter into the rumen. Power is provided by a heavy-duty 3-V lithium battery. The acceleration is probed at 1-s intervals and in a ±2-G range with 10-bit resolution. Transmissions are in the 429-MHz band. This frequency band does not require any license in Japan, but the maximum effective radiated power is restricted to 12.14 dBm. The battery had a mean life of 1 month when measurements of 3-axis acceleration were transmitted continuously every 1 s. This system utilizes a collar repeater to relay the transmissions to receiver that stores data on a cloud-connected server or a personal computer with special software. Communication distance from the collar repeater on the cow to the receiver was about 60 m.
Measurement acceleration data and ruminal motility
Acceleration data from the bolus sensor were transmitted to the receiver in real time. Three-axis acceleration data were recorded to a personal computer with special software connected the receiver. Sampling rates for acceleration was 1 Hz. In terms of three-axis acceleration, the x and z axes indicate the radial acceleration of the probe, and the y axis indicates vertical acceleration. Acceleration (mG) and peak number of accelerations (times/min) from the bolus sensor were calculated as mean values for 10 min. The baseline of the y-axis was kept level, but baselines of the x- and z-axes followed a large curve over the day. The probe was in an almost horizontal position with respect to the longitudinal axis. The basic movement of acceleration of the y-axis showed a very distinct vertical pattern occurring roughly once per minute, with a duration of approximately 8 s, and displaying two local maxima at around 500 mG. On the other hand, acceleration of the x- and z-axes displayed indistinct vertical patterns.
Ruminal motility in cattle was measured using strain gauge force transducers (Arai et al. 2019). Briefly, the force transducer used in this study measured 18 × 35 mm and contained a strain gauge. The force transducer was covered with silicone. Four cattle were sedated intramuscularly with xylazine hydrochloride (0.05 mg/kg). After 10 min, local anaesthesia was applied to the left paralumbar fossa by intramuscular infusion of procaine hydrochloride (80~100 ml/body). The force transducer was sutured to the serosa of the dorsal sac of the rumen, then the lead wires were exteriorized through the abdominal wall. These the wires from the force transducer were then subcutaneously tunnelled and pulled out through the skin. The cattle were injected intramuscularly with antibiotics (10 mg/kg, ampicillin sodium) after the surgery. Ruminal motility was recorded on a polygraph (MP100A; BIOPAC Systems Inc., Santa Barbara, CA, USA) by connecting the lead wires of the transducers to the connecting cables from the amplifiers (DA100; BIOPAC Systems Inc.), then digitised by analogue-to-digital converter, and continuously recorded on a computer for analysis.
Relationship between ruminal motility and acceleration data
We examined the correlation between ruminal motility by force transducer and acceleration by bolus sensor. The bolus sensor was orally administered using a custom-made gastric tube without surgery. The location of the bolus sensor in the reticulum was confirmed by the movement pattern of acceleration (Andersson et al. 2018). A force transducer was sutured to the dorsal sac of the rumen in surgery. Measurements using the force transducer and bolus sensor in the experiment were carried out with cattle 7 days after surgery. Ruminal motility by force transducer and acceleration by bolus sensor were recorded continuously and simultaneously throughout the trial.
Figure 4 shows measurement results of ruminal motility by force transducer and acceleration data by bolus sensor. The waveform of ruminal motility by force transducer and that of acceleration by bolus sensor appeared to largely correspond. In addition, a significant positive correlation was observed between amplitude of ruminal contraction by force transducer and acceleration by bolus sensor. A significant positive correlation was observed between frequency of ruminal contraction by force transducer and peak number of accelerations by bolus sensor. Contraction of the dorsal sac of the rumen and acceleration signals in the reticulum thus occurred at practically the same time. This phenomenon was considered to represent the flow of ruminal contents occurring with compression of the dorsal sac of the rumen pushing and moving the bolus sensor within the reticulum. Braun et al. reported the duration of contraction of the dorsal sac of the rumen as 8.2 ± 1.04 s (Braun and Schweizer 2015). In the present study, duration of acceleration of the bolus sensor was about 8 s. Movement of the dorsal sac of the rumen and acceleration of digesta in the reticulum thus appeared largely correspondent.
The general pattern of reticuloruminal motility consists of regular contraction sequences (Constable et al. 1990, Steller and Stevens 1966) that successively engage the various parts of the reticulum and rumen. There are primary and secondary contraction cycles, with the former serving primarily to mix ingesta, support optimal microbial fermentation and transport ingesta to the omasum, whereas the latter are involved in eructation of gas (Constable et al. 1990). The primary cycles starts with biphasic reticular contraction, followed immediately by contraction of the ruminal atrium. The dorsal sac of the rumen, the caudodorsal blind sac and the left longitudinal groove contract at practically the same time, after which contraction of the caudoventral blind sac and ventral sac of the rumen occurs. The secondary contraction cycle does not involve the reticulum and ruminal atrium and consists of contraction of the dorsal sac and then the ventral sac of the rumen (Braun and Schweizer 2015, Constable et al. 1990). In the present study, acceleration of the bolus sensor generally displayed two local maxima. Since the bolus sensor was located in the reticulum, these local maxima may reflect biphasic reticular contraction. The major waveform of acceleration by bolus sensor probably indicates the flow of ruminal contents occurring by compression of the dorsal sac of the rumen, because a significant positive correlation was observed between acceleration and ruminal motility.
Detection of ruminal atony by bolus sensor
We examined the effect of ruminal motility and acceleration after intramuscular administration of xylazine hydrochloride (0.05 mg/kg; Bayer, Leverkusen, Germany) in cattle as a single dose at 12:00. Measurement data of ruminal motility and acceleration were collected for 120 min before and after administration of xylazine hydrochloride.
Ruminal atony occurred at about 10 min after administration of xylazine hydrochloride. This ruminal atony was detected almost synchronously by bolus sensor and force transducer. As an α2-adrenoreceptor agonist, xylazine is often used in cattle as a sedative. The pharmacological effects of xylazine include excessive depression of the central nervous system (CNS), ruminal atony and bradycardia (Thompson et al. 1991). In the case of disorders of ruminal motility, general anaesthesia and diseases at any sites that produce pain or fever can inhibit the hindbrain reflex centres responsible for evoking primary and secondary cycle contractions of the reticulorumen (Leek 1983). Clinical cases of ruminal stasis should be interpreted as being caused by either depression of the gastric centres, or a lack of excitatory reflex inputs, or an increase in inhibitory reflex inputs, or blockade of the motor pathways as in hypocalcaemic animals, but probably not in the case of vagus indigestion. Besides these specific reflex inputs, the gastric centres are influenced by nervous activity in other parts of the CNS (Leek 1983).
In addition, ruminal tympany (bloat) occurred after ruminal atony. This sign is of particular significance in fattening beef cattle, but may also be a problem in dairy herds on high concentrate rations (Enemark 2008). In healthy cattle, gaseous distension of the reticulorumen reflex elicits an increase in the force and frequency of secondary cycles, evokes opening of the cardia and initiates other respiratory, oesophageal, pharyngeal and buccal actions in the eructation sequence. Normally the cardiac region is cleared when the dorsal ruminal sac contractions of the secondary cycles force the gas layer forward, thereby depressing the fluid level below the level of cardia. In the case of systemic disease where ruminal hypomotility or stasis are secondary features, a persistent, mild bloat may be evident. This is because although the fermentation rate is probably low, weak ruminal motility may be inadequate to move the gas layer and clear the cardia (Leek 1983). The bolus sensor could evaluate ruminal motility such as frequency, amplitude and duration of hypomotility. Evaluation of ruminal motility using the bolus sensor may be useful as a non-invasive method of investigating reticuloruminal stasis in cows with ruminal tympany, acidosis, endotoxaemia, fever and hypocalcaemia (Andersen 2003, Daniel 1983, Leek 1983).
CONCLUSION
Although the probe can perform its intended tasks well in the short term, as demonstrated by this 1-month trial, two primary concerns need to be addressed before this system can be made commercially available. First, the size of the probe should be further reduced. The probe can be administered orally in the current form, but is still large and may prove uncomfortable for the animal. The size-limiting part is the pH sensing assembly and a smaller alternative thus needs to be found or developed. Second, again related to the pH sensor, are concerns regarding the long-term stability of pH measurements. As it stands, the probe can likely detect rapid changes in pH for the duration of its lifetime, but detection of slow changes such as those that would presumably occur if the diet is optimized over the course of several weeks might not be possible. Increasing the stability of the pH sensor is therefore desirable. The bolus sensor could be orally administered without surgery, and was continuously retained in the reticulum. A significant positive correlation was observed between ruminal contraction by force transducer and acceleration by bolus sensor. Contraction of the dorsal sac of the rumen and acceleration signals in the reticulum occurred at practically the same time. The bolus sensor could also detect ruminal atony after xylazine administration. We therefore suggest that the bolus-type wireless sensor may be useful for measuring ruminal motility and detecting dysfunction of the rumen such as ruminal atony and ruminal tympany.
ACKNOWLEDGMENT
This research was supported by a grant (The Research Project for The Future Agricultural Production Utilizing Artificial Intelligence; grant no. ai01) from the Project of the Bio-oriented Technology Research Advancement Institution, NARO.
REFERENCES
Alzahal, O., Alzahal, H., Steele M.A., Van Schaik, M., Kyriazakis, I., Duffield, T.F. and McBride, B.W. 2011. The use of a radiotelemetric ruminal bolus to detect body temperature changes in lactating dairy cattle. J Dairy Sci. 94: 3568-3574.
Andersen, P.H. 2003. Bovine endotoxicosis-some aspects of relevance to production diseases. A review. Acta.Vet. Scand. 98: 141-155.
Andersson, L.M., Arai, S. and Okada, H. 2018. Orally administrable wireless activity and pH probe for cattle reticulum. Sens. Mather. 30: 3029-3038.
Arai, S., Haritani, M., Sawada, H. and Kimura, K. 2019. Effect of mosapride on ruminal motility in cattle. J. Vet. Med. Sci. 81: 1017-1020.
Bewley, J. M., Grott, M. W., Einstein, M. E. and Schutz, M. M. 2008. Impact of intake water temperatures on reticular temperatures of lactating dairy cows. J. Dairy Sci. 91: 3880-3887.
Bligh, J. and Heal, J.W. 1974. Use of radio-telemetry in study of animal physiology. Proc. Nutr. Soc. 33: 173-181.
Braun, U. and Schweizer, A. 2015. Ultrasonographic assessment of reticuloruminal motility in 45 cows. Schweiz. Arch. Tierheilkd. 157: 87-95.
Constable, P. D., Hoffsis, G. F. and Rings, D. M. 1990. The reticulorumen: Normal and abnormal motor function. Part I. Primary contraction cycle. Comp. Cont. Educ. Pract. Vet. 12: 1008-1014.
Constable, P. D., Hoffsis, G. F. and Rings, D. M. 1990. The reticulorumen: Normal and abnormal motor function. Part II. Secondary contraction cycles, rumination, and esophageal groove closure. Comp. Cont. Educ. Pract. Vet. 12: 1169-1174.
Daniel, R. C. W. 1983. Motility of the rumen and abomasum during hypocalcaemia. Can. J. Comp. Med. 47: 276-280.
DePeters, E.J. and George, L.W. 2014. Rumen transfaunation. Immunol. Lett. 162: 69-76.
Duffield, T., Plaizier, J. C., Fairfield, A., Bagg, R., Vessie, G., Dick, P., Wilson, J., Aramini, J. and McBride, B. 2004. Comparison of techniques for measurement of rumen pH in lactating dairy cows. J. Dairy Sci. 87: 59-66.
Egert, A. M., Klots, J. L., McLeod, K. R. and Harmon, D. L. 2014. Development of a methodology to measure the effect of ergot alkaloids on forestomach motility using real-time wireless telemetry. Front Chemi. 2(90): 1-8.
Enemark, J. M. D. 2008. The monitoring, prevention and treatment of sub-acute ruminal acidosis (SARA): A review. Vet. J. 176: 32-43.
Falk, M., Munger, A. and Dohme-Meier, F. 2015. Technical note: A comparison of reticular and ruminal pH monitored continuously with 2 measurement systems at different weeks of early lactation. J. Dairy Sci. 99: 1951-1955.
Hamilton, A.W., Davison, C., Tachtazis, C., Andonovic, I., Michie, C., Ferguson, H.J. Somerville, L. and Jonsson N.N. 2019. Identification of the rumination in cattle using support vector machines with motion-sensitive bolus sensors. Sensors 19, 1165.
Klevenhusen, F., Pourazad, P., Wetzels, S. U., Qumar, M., Khol-Parisini, A. and Zebeli, Q. 2014. Technical note: Evaluation of a real-time wireless pH measurement system relative to intraruminal differences of digesta in dairy cattle. J. Anim. Sci. 92: 5635-5639.
Leek, B.F. 1983. Clinical diseases of the rumen: a physiologist’s view. Vet. Rec. 113: 10-14.
Madan, J., Gupta, M., Rose, M.K., Sindhu, S. and Kar, D. 2013. Metabolic alterations in buffaloes suffering from digestive disorders. Haryana Vet. 52: 71-72.
Mottram, T., Lowe, J., McGowan, M. and Phillips, N. 2008. Technical note: A wireless telemetric method of monitoring clinical acidosis in dairy cows. Comput. Electron. Agric. 64: 45-48.
Nogami, H., Arai, S., Okada, H., Zhan, L. and Itoh, T. 2017. Minimized bolus-type wireless sensor node with a built-in three-axis acceleration meter for monitoring a cow’s rumen condition. Sensors 17, 687.
Rose-Dye, T.K., Burciaga-Robles, L.O., Krehbiel, C.R., Step, D.L., Fulton, R.W., Confer, A.W. and Richards, C.J. 2011. Rumen temperature change monitored with remote rumen temperature boluses after challenges with bovine viral diarrhea virus and Mannheimia haemolytica. J. Anim. Sci. 89: 1193-1200.
Sato, S., Ikeda, A., Tsuchiya, Y., Ikuta, K., Murayama, I., Kanehira, M., Okada, K. and Mizuguchi, H. 2012. Diagnosis of subacute ruminal acidosis (SARA) by continuous reticular pH measurements in cows. Vet. Res. Commun. 36: 201-205.
Sato, S., Kimura, A., Anan, T., Yamagishi, N., Okada, K., Mizuguchi, H. and Ito, K. 2012. A radio transmission pH measurement system for continuous evaluation of fluid pH in the rumen of cows. Vet. Res. Commun. 36: 85-89.
Stellers, A. F. and Stevens, C. E. 1966. Motor functions of the ruminant forestomach. Physiol. Rev. 46: 634-661.
Thompson, J. R., Kersting, K. W. and Hsu, W. H. 1991. Antagonistic effect of atipamezole on xylazine-induced sedation, bradycardia, and ruminal stony in calves. Am. J. Vet. Res. 52: 1265-1268.
Reviewed, edited and uploaded: November 25, 2019