The Challenges of Achieving Optimal Balance between Crop Productivity and Sustainability in a Changing Climate[1]*
Holger Meinke1,2
1 Tasmanian Institute of Agriculture, University of Tasmania, Hobart, TAS 7001, Australia
2 Centre for Crop Systems Analysis, Wageningen University, Netherlands
e-mail: holger.meinke@utas.edu.au
ABSTRACT
Change creates risks and opportunities. We can try to prevent it, resist it or adapt to it. The recent rate of environmental change has created urgency for well-planned adaptation action. It might be timely to consider the concept of ‘adaptation science’ as a process that generates the insights needed to affect changes in agricultural systems towards increased adaptive capacity and performance. This requires quantitative assessments of threats, risks and opportunities. It also requires appreciation and understanding of the socio-economic drivers of change. For adaptation science to have high social impact it needs to be salient, credible and legitimate.
Keywords: risk management, climate change, adaptation science, systems modeling
Introduction
We live in a world of increasingly rapid changes. Change creates risks and opportunities. Change is also a simple fact of life. Nature’s response to change is evolution. To successfully tackle the challenges of the 21st century – feeding over nine billion people sustainably - we need to ‘turbo-charge’ evolutionary processes; we need to become proactive in order to keep abreast of the continuously accelerating change process. We need to actively adapt.
When forces of change begin to exert pressure, there are only 4 possible responses:
-
We can ignore them;
-
we can try to resist;
-
we can attempt to mitigate against these forces or;
-
we can adapt.
Whatever we do – or whatever we don’t do – in response to this pressure is our choice; even inaction is a choice. And ultimately, we are all accountable for our choices. This means that we are inevitably part of the change process in a constant struggle to improve the human condition. We cannot escape it. Nobody can.
Agricultural science in all its complexity needs to play an active role in this rapidly changing world. Specifically, I want to highlight the importance of a systems analytical approach to ensure profitable and ecologically sound crop production. I want to highlight the relevance of such research for our societies. Many of my examples will refer to climate as a key driver of change in agricultural systems. Even though I will focus on climate risks, the approach I am suggesting is about risk management in agriculture generally, regardless of the underlying driver. However, given the importance of climate risks - and lately of course the increasing need to adapt to a changing climate – it is appropriate to focus on climate risks.
DISCUSSION
I have already mentioned the four possible responses to change: inaction, denial, mitigation and adaptation. When it comes to global and climate change, inaction and denial are no longer acceptable responses. By now we know far too much about the processes responsible for climate change; we also know far too much about their consequences to simply sit back and do nothing. It would be immoral to leave all the consequences of our affluent lifestyles simply to future generations to deal with. I fear that they will judge us harshly anyhow; and rightly so.
This leaves us with mitigation and adaptation. Now mitigation is most effective when action is taken early in order to prevent the system to evolve to a stage where drastic changes will occur. I think it is too late for this. Just to re-enforce this point, here is a very famous diagram, which I am sure you all have seen before in one form or another. It is a continuation of Keeling’s original atmospheric CO2 concentration curve (Fig. 1).
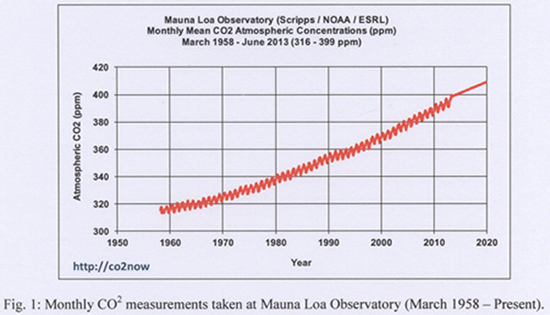
The beginning of Keeling’s CO2 measurements coincides with the year of my birth. The measurements show that in my lifetime alone, CO2 has increased by 85ppm, from 315ppm to nearly 400ppm today. Compared to the pre-industrial levels of around 280ppm, CO2 concentrations have now increased by 120ppm or 43%. We will be well into the 400ppm range by the time I will retire. Not too many statisticians would argue with me about this extrapolation. I regard this as a fairly certain; it is a foreseeable event.
There is something else that is close to certain: these increases in greenhouse gasses (GHG) are already impacting on our climate and climate sensitive sectors of our society. At a global scale we can see the impacts, many of them, from melting ice caps, retreating glaciers to the extinction of species, because there is no mountain high enough for them to escape to. We monitor and measure species diversity and we know that biodiversity is seriously under threat (for many reasons, not just climate change). But from here on, any certainty quickly dissipates.
Now let’s have a look at a second famous diagram. This is work by Rahmstorf from 2007 (Fig. 2). It shows two different measurements of annual, global temperature data with the IPCC projections for the different emission scenarios superimposed. I only want to point out one aspect of this diagram: it shows that ever since the IPCC began issuing projections, sometime in the mid 1990s, we have been tracking at the upper end of the scenario envelope.
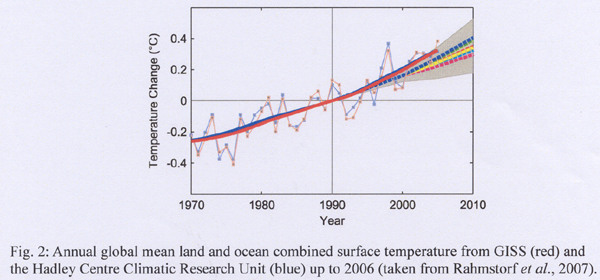
And yes, I know, every single piece of scientific evidence is contentious, can be disputed. The point is: it is not the individual pieces of evidence that matter. What matters is that collectively the case is so compelling that inaction amounts to malicious negligence. Just a few very selective examples should suffice (Fig. 3):
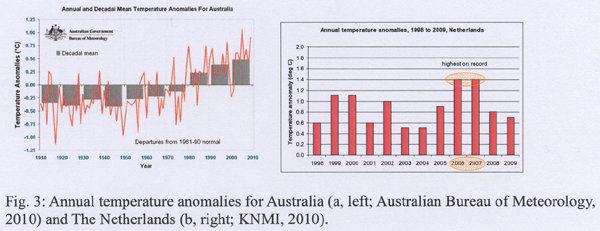
Consider the rapidly melting ice caps and glaciers; remember the European heat wave of 2003; or the fact that 2012 was again amongst the 10 warmest years on record with sea ice extent in the Arctic reaching a record low. In fact, the Arctic continued to warm at about twice the rate compared with lower latitudes, while sea levels are at a record high, increasing at an average rate of 3.2 (+/- 0.4) mm (NOAA, 2013). And in January 2013, Hobart, Australia’s most southern capital city on an island surrounded by the Southern Ocean reached 43oC for the first time ever.
Or consider the Australian floods and bush fires of February 2009 that killed 200 people – these events occurred simultaneously, in different parts of the country. Fig. 4 shows the land surface temperature anomalies in the week leading up to these disasters; it clearly shows the intensity of these two contrasting extreme events.
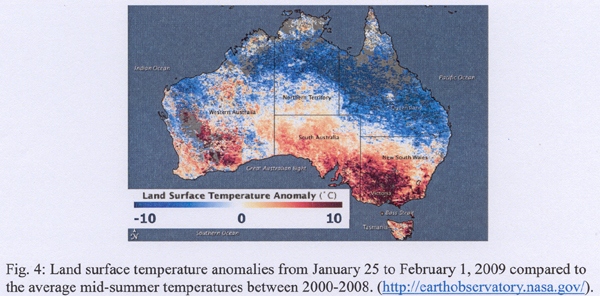
Meanwhile many of our natural ecosystems such as coral reefs - places of incredible bio-diversity and beauty - are destroyed at alarming rates by increasing ocean temperatures and acidity. In short: the accumulated evidence is overwhelming.
Which brings me back to my earlier comments: Mitigation, that is the avoidance of processes that lead to climate change, is most effective and cheapest when taken early. This means that we should have begun decarbonising our economies way back in the 1960s when CO2 levels were still below 350ppm. We chose not to do this.
The choice not to act has two consequences: Firstly, it increases the urgency for mitigation action to avoid further substantial damage and secondly it increases the urgency to adapt as a certain amount of climate change is now unavoidable (Howden et al., 2007).
Although mitigation is politically extremely difficult, scientifically we can competently approach it: We need to reduce our GHG emissions by decarbonising our economies. This is a paradise for research industries and it creates much room for disciplinary-based innovations: from alternative energy sources to carbon capture such as clean coal technologies and underground CO2 storage facilities; reducing methane emissions from rice paddies or livestock (just think about the new breed of sheep that burp less) to riding your bike instead of using the car. While there is one goal, namely the reduction of GHG emissions, the means of achieving this goal are diverse and varied – the more, the better (Table 1).
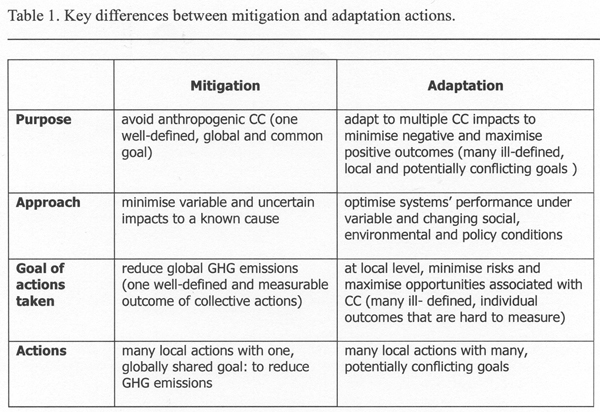
Yet, politically mitigation is difficult; very difficult. In Australia we are in the middle of an election campaign where our very modest carbon tax is a very hot political issue. But if we think that mitigation is difficult, with adaptation, things get even more complicated as there is no single, agreed goal of what adaptation is supposed to achieve. The issue of competing claims and conflict agendas looms largely in the adaptation debate. Questions need to be answered such as: Who is adapting to what and with which consequences? And the issue of scale suddenly raises its head even more than before.
While reducing GHG emissions at any scale anywhere contributes to the ‘global good’, which is the overarching goal of mitigation, adaptation actions are largely independent, local and very context-specific. Hence, compared to mitigation, real adaptation actions are even harder to agree on and incredibly complex to achieve. Adaptation actions are highly contestable: what works well for certain sections of a community, can be to the detriment of others (Nelson et al., 2010).
Many people still object to the fact that something as dangerous as humanly induced climate change can actually result in opportunities and benefits for certain sectors and groups of individuals. This leaves science with a big conundrum: how can we best apply our narrow and very specific scientific knowledge to the issues of adaptation? How can we capitalise on opportunities without causing further damage?
Although adaptation is nothing new, the scientific adaptation agenda is – until only a few years ago, adaptation to climate change was seen by many as an issue to be avoided because it gave the impression of inevitability, of a system out of our control. It was regarded as politically dangerous and damaging. However, the evidence and urgency has now increased to such an extent that even most of the die-hards acknowledge that the time for adaptation action is now.
I want to briefly put adaptation in relation to agriculture in historical context. For this I will use 3 examples:
1) The Norse
Exactly 1027 years ago, in 986, Erik the Red led a group of Viking families from Iceland to settle in the southern parts of Greenland. 422 years later, in 1408, a wedding performed in the Hvalsey Church (Fig. 5) is the last recorded event before all settlements were again abandoned (Lundberg, 1999). What went wrong?
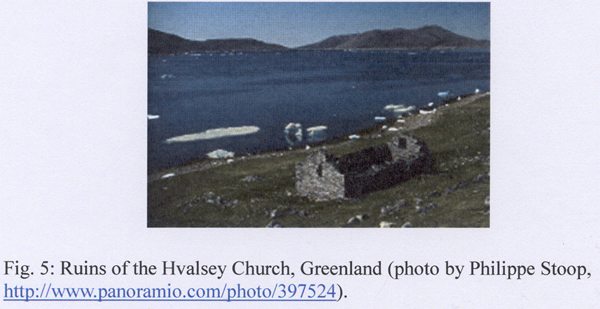
At the time of settlement, Greenland’s climate was considerably warmer than during the 20th century. The new settlers were farmers engaged in animal husbandry and cropping, who managed to accumulate enough food and fodder during the short summer season for a reasonable living.
However, the mild climatic conditions were fairly short-lived in geologic terms and by about 1200, progressively cooler conditions made life extremely difficult. In some years, no supply ships were able to reach Greenland due to persistent sea ice preventing the passage of ships (Lundberg, 1999). By the early 1300s, significant changes in temperature are recorded in such diverse materials as glacier ice, fossil vegetation and pollen deposited in lake sediments, chemical signatures in isotopic composition of sea sediments, animal and human bones (Fricke et al., 1995). This cooling climate led to agricultural practices that became progressively less sustainable and, as a consequence, depleted Greenland of its natural capital - its grasslands, animal resources and cropping opportunities.
For instance, fossil pollen indicates that the expansion of grasslands into increasingly marginal areas led to erosion. Land degradation forced people to push production even further until it exceeded the regenerative capacity of Greenland's environment, leading to a downward spiral of productivity and ultimately starvation (Panagiotakopulu and Buckland, 2007). Isotopic evidence from ice cores indicates that the 14th century was the coldest known in Greenland for 700 years.
Today, the arctic is warming at twice the rate of the rest of the world and agriculture is predicted to increase significantly in a region so far free of many pests and diseases (Jensen et al., 2009). Greenlanders are proud of the fact that they are again engaged in agriculture: home-grown potatoes, broccoli and strawberries have recently become part of the Greenland diet, while options for growing barely on a larger scale are being explored (Fig. 6). Greenland is capitalising on some of the arising opportunities from climate change.
.jpg)
2) The Potato Eaters
Let me move on to another example: This evocative painting by Vincent van Gogh (Fig. 7) is called ‘The Potato Eaters’. Vincent painted it in 1882 and it is a classical example of human adaptive capacity. Very skillfully it symbolises multilevel adaptation: while the problem was largely climate induced poverty and famine – caused by natural climate variability – one solution was the growing and eating of potatoes.
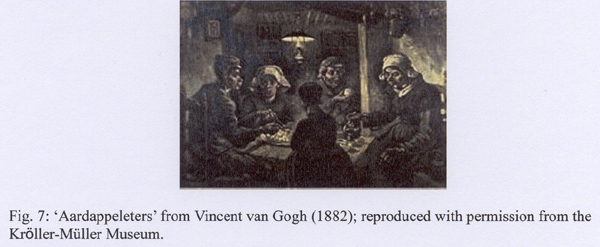
Potatoes were first introduced into Europe in the mid 16th century, just at the beginning of the ‘little ice age’ when crop yields generally were declining. The key innovation was that potatoes provided 2-3 times more food per unit land compared to cereals such as wheat. The introduction of potatoes into Estonia in the 1840s put an end to frequently recurring famines that had killed over 75,000 people in Estonia alone (Tannberg et al., 2000).
By the mid 19th century potatoes were therefore considered the ‘bread of the poor’, a staple food and a reliable substitute for wheat. So out of necessity, poor European farmers adapted their cropping systems as well as their eating habits; adaptation is always multi-faceted.
This is risk management in action – risk management defined as the systematic process of identifying, analysing and responding to risk. It includes maximising the probability and consequences of positive events and minimising those of adverse events. Good risk management seizes opportunities without ideological biases.
The recent pace of global change has added an additional component of urgency for planned risk management and proactive adaptation. It is now time to address this issue more systematically, particularly when it comes to agriculture.
This brings me to the emerging field of ‘adaptation science’ (Meinke et al., 2009): Adaptation science is not a new science – it is simply a different way of conducting science. The traditional, reductionist approach to science has a tendency to create islands of knowledge in a sea of ignorance. Traditional science focuses much more on analysis of scientific inputs rather than on the synthesis of socially relevant outcomes.
Adaptation science, on the other hand, is very much outcome, solution oriented. This new way of scientific inquiry differs from the traditional approach of ‘science for adaptation’ by attempting to provide societal relevant solutions rather than just more disciplinary based data. Adaptation science is a problem focused and solution oriented science paradigm that, without prejudice, tries to combine societal relevance with scientific excellence. It has a lot in common with the notion of ‘adaptive management’, an ecological approach to action increasingly popular within the conservation movement. But more of this later.
Given the urgency created by the accelerating pace of climate change, climate risks serve as a good example of how adaptation science might bring together scientific knowledge from many disciplines in order to manage our limited resources more sustainably and profitably. And although we cannot predict with certainty what is going to happen, many of the problems that are in-store for us are very well foreseeable.
The concept of foreseeability is key here: the best example I can think of that distinguishes foreseeable events from predictions is when you run a red traffic light at a busy intersection. I cannot predict that you will have an accident, but I can foresee that your chances of having an accident will be dramatically increased. Foreseeability is a legal concept that dictates that if a disaster or an accident is foreseeable, but the operator of the system does not take necessary precautionary action he or she will be liable. Future generations will hold us to it.
Adaptation science takes such risk management principles seriously. Science today offers us the unique opportunity to (a) better understand causes and consequences of our actions and (b) learn from history, by being better prepared and willing to adapt.
Let me give you a final historical example to underline the need to adapt, this time from my home continent, Australia:
3) South Australian settlers in the late 19th century
South Australia’s ‘Goyder Line’ is an icon in Australia’s agricultural history. The line was first drawn by George Goyder, a geographer extraordinaire and South Australia’s surveyor-general at the time.
Driven by a deep, intuitive understanding of the role of rainfall variability as a key driver of risk for wheat farming in low rainfall regions, Goyder set out in 1865 to determine 'the line of demarcation between the portion of the country where the rainfall has extended and that where the drought prevails' (Government of South Australian, 2010).
George Goyder went out on a horse, examined the natural system, particularly plants and soils, and drew inferences from this knowledge. Based on this, he drew a line on a map to highlight the areas where, in his opinion, cropping should never be attempted (Fig. 8). The line runs roughly west to east across the mid-north of SA from the edge of the desert, the Nullarbor Plain, to the Victorian border (between 33o and 34o S; ABC, 2009).
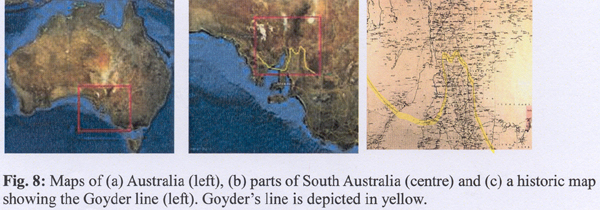
Goyder was a visionary and one of the very first people to perceive the degree and impact of Australia’s highly variable rainfall. He postulated that north of his line, which equates roughly to the 300mm annual rainfall zone, rainfall was too low and erratic for viable wheat production. These days we use simulation models to arrive at such conclusions. Goyder conducted this systems analysis in his head!
Just a few years later, however, Goyder’s line became highly controversial when a series of wet years resulted in farmers establishing properties way north of his line. He was attacked in parliament, ridiculed in the press. At public meetings, people called for Goyder and his line to be thrown out of the colony (ABC, 2009). When more variable and drier conditions returned yet again in the late 1880s nearly all of the new farms were abandoned resulting in considerable hardship.
Goyder’s line had an enormous influence on the Australian psyche and rural settlement policies. He was at least one century ahead of his time in understanding rainfall variability and its impact on natural ecosystems and agricultural productivity. Since then generations of Australian farmers have learned at their peril to ignore climate variability and its consequences on farm businesses. As a consequence, Australia has probably one of the most ‘climate aware’ agricultural sectors in the world.
Lessons learned?
The experience of the Norse, the early Australian settlers and poor European farmers should be sufficiently convincing that adaptation science – a more formal, transdisciplinary approach to scientific inquiry – is urgently needed. But how will it work? What do we need to do differently when compared to what we already do?
In the examples I provided, everyone would have benefited from the knowledge-generating technologies that are at our disposal today. For agriculture ‘creating hindsight in advance’ has only become possible based on the scientific breakthroughs in modelling complex systems. This modelling work (i.e. ‘system thinking’) has its origin at Wageningen University in The Netherlands, where Prof. Cees de Wit first introduced systems theory and simulation modelling in the 1950s and 60s. The global science community continues to build on the foundations de Wit created.
Today we use models to create a new type of knowledge that is similar to the conventional, empirical knowledge that decision makers usually draw on when managing risks holistically and through intuition (e.g. Keating et al., 2003; Meinke et al. 2009). This provides the cornerstones for adaptation science, which draws heavily on such knowledge-generating technologies. The ability to analyse and simulate complex systems is essential for ‘creating hindsight in advance’.
I already outlined the need for adaptation science; I will now argue that this way of doing science is highly context-specific and needs to be in itself ‘adaptive’. This might even include a requirement for new, different institutions and the way they interact.
So how will adaptation science work? For this I propose a 4-step approach (Fig. 9; Meinke et al., 2009):
-
agree on the problem domain such as ‘securing food for the poor’, ‘improve the efficiency of water use’ or ‘minimise crop losses due to weed infestation’, to name just 3 of an unlimited number of examples;
-
agree on the transdisciplinary scientific objectives, i.e. what mix of disciplinary knowledge is required to make the best possible scientific contribution towards solving the problem;
-
agree on partnerships and governance of the team that are supposed to develop the solutions; in most cases this will be a community of practice, i.e. a group of people – including scientists – from many different domains who share a common interest in solving specific problems;
-
do the work.
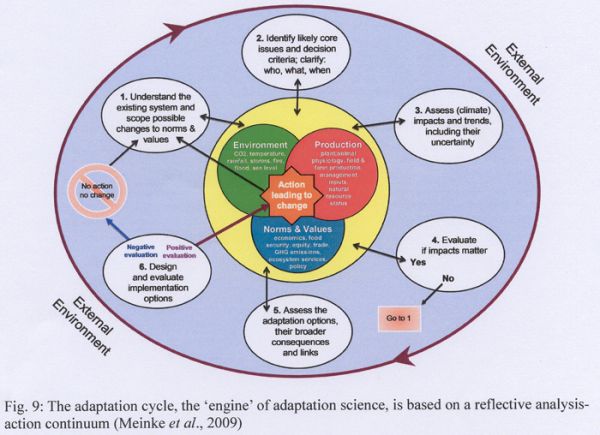
Let us for now assume that we have established such a community of practice around the issue of climate risk in agriculture. One of the first hurdles we have to overcome, even before we turn this into a truly transdisciplinary community, is breaking down the barriers within disciplines. While much of the talk is about climate change, the real issue from a farmer’s perspective is managing scarce resources such as land, water and labour within the context of a risky environment. What we often forget is that climate change will be delivered to the farm gate – free of charge – by climate variability (Meinke and Stone, 2005). As one of my colleagues often quips: ‘Who destroyed the sand castle? Was it the wave or was it the tide?’ (Fig. 10). This is a powerful analogy that stresses how existing climate variability rides on a slowly rising tide. Unless we help the rural sectors in both developing AND developed countries to proactively adapt to climate variability and change, we fail in our responsibility as scientists.
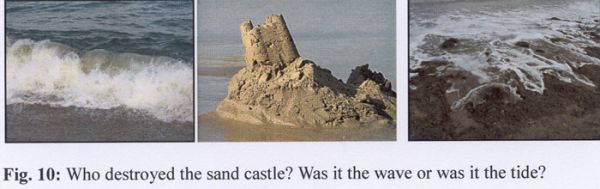
There is no doubt that plants, and hence crops, will remain the single most important objects in the quest to solve the problems of our planet. Without plants there will be no life. A systems analytical approach is uniquely placed to provide the underpinning science for adaptation actions. We can now develop and apply the quantitative modelling tools that are needed to assess the value of different adaptation options for agricultural production systems.
Good crop modelling at the scientific forefront of understanding how plants and plant systems function. To better understand and assess the function of these systems, we use simulation modelling as one of our core technologies. Ideally a good modelling team should be multi-disciplinary including scientists comprising plant physiologists, crop physiologists, cropping systems experts, ecologists, modellers and climate scientists – to name just some of the key disciplines that are essential for problem solving in agriculture.
Of course, in spite of being a multidisciplinary team, often additional expertise is needed to solve real life problems. Hence, global collaboration is the key to a group’s success. Collaborative networks should be extensive and reach all corners of the earth. This is one of the reasons why I am here today.
Let me give you an example that might resonate with you:
Rice is the world’s most important staple food; 91% of all rice is produced in Asia. There is limited scope to increase production due to competing claims on scarce resources such as water, land and labour. Increasing temperatures, more frequent droughts, anticipated loss of productive estuaries caused by rising sea levels and more frequent and severe storms further compounds these problems. This creates urgency for rice-based production systems to produce more rice with less water, less land or less labour; in other words: we need to increase these various efficiencies, which are also referred to as ‘eco-efficiencies’.
In his classic paper on “Resource Use Efficiency in Agriculture”, Cees de Wit (1992) explored these eco-efficiencies. His paper sparked intense scientific debate, which, unfortunately, I don’t have time to detail here. There is agreement, however, that eco-efficiencies are highly contextual. To understand these complexities, we need formal, quantitative methods that help us to decide, which efficiency improvement to target for maximum gain. For this, an efficiency frontier framework can provide valuable guidance when making research investment decisions (Fig. 11; Keating et al., 2010):
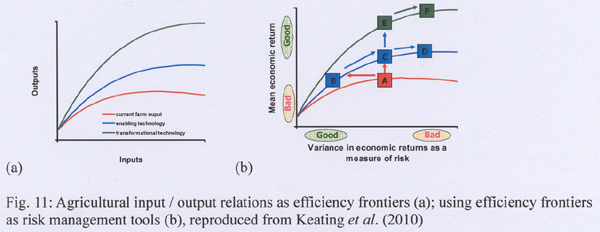
The diagrams are stylised presentations of input / output relations for agricultural production. They describe general responses to inputs at various levels of intensity and co-limitations. Particularly under severely limited conditions, this approach can help to determine the most appropriate starting point in order to increase productivity and efficiency of production. How do we know which type of technology might be best suited for such improvements? To answer this question, we consider 3 different levels of input / output relationships in a developing country context:
-
current farm output – usually under severe resource limitation; here we often find that increasing the amount of a single production factor does not significantly raise production outputs;
-
enabling technologies – that is technologies that are readily available and could at least theoretically be supplied, such as fertiliser combined with better management of pest and diseases; and
-
transformational technologies – that is cutting edge science, such as ‘turbo charging’ the photosynthetic process or completely new management practices.
Let us explore how we can use this framework operationally:
Increasing inputs can increase outputs, but it usually also increases output variability, particularly when climate is highly variable. Therefore, the variance in economic returns can be used as a surrogate measure for risk. On the x-axis of Fig. 11b we replaced inputs with a measure of risk, namely the variance in economic returns of a certain action. The y-axis shows the longterm or mean economic return of a certain action. I will now briefly demonstrate how such efficiency frontiers can be used as risk management tools:
-
We can use enabling technologies to either maintain current productivity but reduce risk by moving from A to B;
-
We can also use enabling technologies to address systems inefficiencies at ‘constant risk’ by moving from A to C; extra income generated through these improvements can now be re-invested.
-
Once we have achieved this, we can move along the new efficiency frontier and make use of the increased responsiveness to inputs by moving from C to D;
-
and finally we can lifting productivity to a new level through transformational technologies (C to E and E to F).
With the help of models, the necessary data for such response functions can be derived and foreseeable events can be probabilistically described (Maia and Meinke, 2010) and then used for a range of stakeholders who can then engage in meaningful dialogues amongst themselves (Fig. 12).
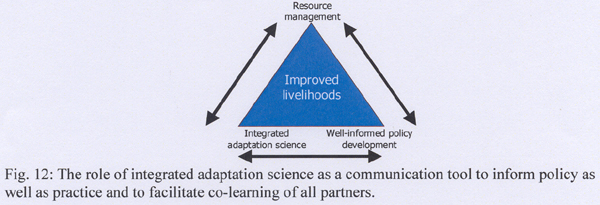
Once this is achieved, the framework can be used to diagnose the most promising pathways for intervention into agricultural systems to improve their performance – either incrementally or via fundamental changes to the system. Used in this way, integrated adaptation science can really help to improve the livelihoods of the most vulnerable people.
So to improve the productivity of rice-based systems, we need to explore all options, incremental improvements and revolutionary change. What works best will depend on the context. We need incremental improvements, for instance better water management based on seasonal climate forecasts, improved infrastructure and adapted varieties. This moves us along or onto the existing efficiency frontier. We also need the transformational changes in places where there is simply not enough irrigation water left.
For rice-based systems in water-scarce environments, this is when aerobic rice – that is rice that is not grown under flooded conditions – comes into play (Fig. 13). While production per unit area will not increase or possibly even slightly decrease under aerobic conditions, water use efficiency gains of more than 100% are clearly achievable. In terms of water use, this will move us onto a new efficiency frontier.
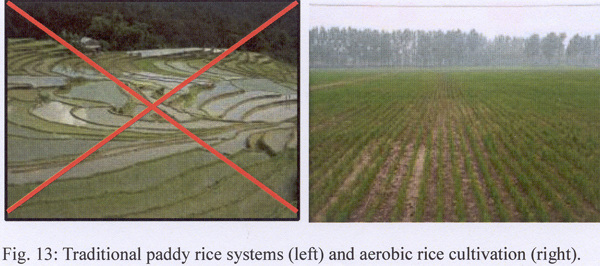
This is ‘adaptation by design’ – a careful analysis of all the options before implementing the most promising ones. Clearly, this requires good modelling skills at all scales that draw strongly on expertise across an extensive network of scientists.
I would rather like to briefly touch on three more points:
Firstly, the importance of teaching and education
The success of a university depends very much on a strong commitment to diversity and true inclusiveness. We must the education of the next generation as our civic duty rather than a privilege that is only accessible to some. The importance of equity and inclusiveness cannot be overrated. This has consequences for our institutions, particularly our universities:
-
we need to use science to ensure equitable access to food, fibre and other resources such as water and
-
we need to fight for access to knowledge by all humans.
The second point I would like to stress is the importance of flexibility, tolerance and self-reflection
We can’t talk about adaptation without adapting ourselves. In fact, this is one of the principles of adaptive governance of which adaptation science is a logical extension. In my group, rather than fixing our structure and our strategic direction, we constantly reflect and review. In a world where the pace of change is rapidly increasing, we must remain responsive to these changing demands. True to the principle of life-long learning, we endeavour to be a responsive and proactive group that constantly re-invents itself rather than defending our past. Following the principles put forward by Hamel and Välikangas (2003), we aim to achieve revolutionary change in lightning-quick, evolutionary steps, but with no calamitous surprises, no convulsive re-organisations, no across-the-board staff changes. In a truly resilient organisation, there should be plenty of excitement, but no trauma. At the very least we should try creating work environments where great ideas and innovation can thrive, but where the direction is clear and shared by all.
And finally: social change
Agriculture has been the engine for social change in human history. Without an abundance of food, there wouldn’t be any change. So isn’t it ironic that rural people and regions are now perceived as the ones most resistant to changes? Maybe having lost control over the forces agriculture created, there is a now tendency to ‘dig in’, circle the wagons. Although this is an understandable reaction, it is not a strategy that will succeed. The global forces are far greater than any resistance can withstand. But there are alternatives:
The 1960s and ‘70s were the decades of the green revolution, based on research-based, technological breakthroughs with all their positive and negative consequences. This was followed by a period of relative calm. Now, with climate change and the emerging pressure on our resource base and rapidly growing populations on our doorstep, we have entered into another type of agricultural revolution: the need to feed a growing population sustainably without increasing our resource use.
Being a serious player in this new round of transformational changes requires knowledge and wisdom. These are commodities that are not sold in 20kg bags. Knowledge is hard to acquire and it takes time and effort. The people who will influence the outcomes of this new revolution will be well-educated, and not just in agricultural practice. Yes, they will understand the principles of farming, but they will also be experts in global finances; they will understand the importance of sustainable practices; they will be effective mangers of human resources and they will shape the inevitable debates about contentious issues. They will combine business acumen with social intelligence. In short: they will be real leaders. And we, the agricultural research community, need to be among them, driving this change.
CONCLUSION
The pace of environmental and social change will accelerate. Social changes will be led by groups and individuals who are highly educated and influential. The best we can hope for is that as many of these change agents as possible will have a solid understanding of agricultural systems and their role in our societies. We need to get amongst them.
Agriculture and Food Systems ARE the new black! Universities have a responsibility to provide the rounded education that the current and future players in this knowledge-intensive game need to succeed. We are in the midst of a knowledge-based revolution and we need to take charge of it. We need to ensure that the next generation of scientific leaders understands agriculture. Tertiary education has never been as important as right now, so now it not the time to pull back and divest from the tertiary sector. ‘Creating an abundance’ of knowledge and wisdom is the best investment we can make. We need productive minds to manage our productive landscapes. We need a productive tertiary sector.
REFERENCES
ABC (Australian Broadcasting Corporation), 2009. http://www.abc.net.au/rn/hindsight/stories/2009/2540163.htm
Australian Bureau of Meteorology, 2010. Annual Australian Climate Statement 2010. http://www.bom.gov.au/announcements/media_releases/climate/change/20100105.shtm
Carlson, M., 2006. History of Medieval Greenland; http://www.personal.utulsa.edu/~marc-carlson/history/grontimebible.html
Fricke, H.C., O'Neil, J.R. and Lynnerup, N. 1995. Oxygen isotope composition of human tooth enamel from medieval Greenland: Linking climate and society. Geology 23, 869-872.
Government of South Australian, 2010. State records of South Australia. http://www.archives.sa.gov.au/exhibits/saonmap/gwgtimeline.html
Hamel, G. and Välikangas, L. 2003. The Quest for Resilience, September 2003, 1-13.
Howden, S.M., Soussana, J-F., Tubiello, F.N., Chhetri, N., Dunlop, M. and Meinke, H., 2007. Adapting agriculture to climate change. PNAS, 104(5), 19691–19696. doi:10.1073/pnas.0701890104.
Jensen, C., Munk, L., de Neergaard, E., Høegh, K. and Stougaard, P., 2009. Biocontrol bacteria isolated from potato fields in Greenland. Climate Change: Global Risks, Challenges and Decisions. IOP Publishing IOP Conf. Series, Earth and Environmental Science 6; doi:10.1088/1755-1307/6/7/372041.
Keating, B.A., Carberry, P.S., Hammer, G.L., Probert, M.E., Robertson, M.J., Holzworth, D., Huth, N.I., Hargreaves, J.N.G., Meinke, H., Hochman, Z., McLean, G., Verburg, K., Snow, V., Dimes, J.P., Silburn, M., Wang, E., Brown, S., Bristow, K.L., Asseng, S., Chapman, S., McCown, R.L., Freebairn, D.M. and Smith, C.J., 2003. An overview of APSIM, a model designed for farming systems simulation. European Journal of Agronomy, 18: 267-288.
Keating, B.A., Carberry, P.S., Bindraban, P., Asseng, S., Meinke, H. and Dixon, J., 2010. Eco-efficient agriculture: concepts, challenges and opportunities. Crop Science, accepted.
KNMI (Koninklijk Nederlands Meteorologisch Instituut), 2010. http://www.knmi.nl/klimatologie/maand_en_seizoensoverzichten/index.html
Lundberg, M., 1999. The history of Greenland. http://explorenorth.com/library/weekly/aa121799.htm
Maia, A.H.N. and Meinke, H., 2010. Probabilistic methods for seasonal forecasting in a changing climate: Cox-type regression models. Int. J. Climatology., in press, doi:10.1002/joc.2042.
Meinke, H., 2010. Adaptation Science for agriculture - solutions for a changing planet. Inaugural lecture, Wageningen University, 14 January 2010, ISBN 987-90-8585-271-1, pp. 34.
Meinke, H. and Stone, R.C., 2005. Seasonal and inter-annual climate forecasting: the new tool for increasing preparedness to climate variability and change in agricultural planning and operations. Climatic Change, 70, 221-253.
Meinke, H., Howden, S.M., Struik, P.C., Nelson, R., Rodriguez, D. and Chapman, S.C., 2009. Adaptation science for agricultural and natural resource management – Urgency and theoretical basis. Current Opinion in Environmental Sustainability, 1, 69–76. doi:10.1016/j.cosust.2009.07.007.
Nelson, R., Kokic, P., Crimp, S, Meinke, H. and Howden, S.M., 2010. The vulnerability of Australian rural communities to climate variability and change: Part I – Conceptualising and measuring vulnerability. Environmental Science and Policy. 13, 8-17. doi:10.1016/j.envsci.2009.09.006.
NOAA, 2013. State of the Climate in 2012: Highlights. http://www.climate.gov/news-features/understanding-climate/state-climate-2012-highlights
Panagiotakopulu, E. and Buckland, P., 2007. Fossil insect evidence for the end of the Western Settlement in Norse Greenland. Naturwissenschaften 94, 300–306; DOI 10.1007/s00114-006-0199-6
Rahmstorf, S., Cazenave, A., Church, J.A., Hansen, J.E., Keeling, R.F., Parker, D.E. and Somerville, R.C.J., 2007. Recent climate observations compared to projections. Science 316, 709.
Tannberg, T., Mäesalu, A., Lukas, T., Laur, M. and Pajur, A. 2000. History of Estonia. Avita, Tallinn, 335 p.
Wit, C.T. de 1992. Resource use efficiency in agriculture. Agric. Systems, 40, 125-151.
Submitted as a keynote paper for the FFTC-TARI (Taiwan Agricultural Research Institute) International Workshop on Strategic Approach to Integrate Practical Technologies for Climate-Smart Crop Production |
[1] * This talk is largely based on my inaugural lecture (Meinke, 2010)
The Challenges of Achieving Optimal Balance between Crop Productivity and Sustainability in a Changing Climate
The Challenges of Achieving Optimal Balance between Crop Productivity and Sustainability in a Changing Climate[1]*
Holger Meinke1,2
1 Tasmanian Institute of Agriculture, University of Tasmania, Hobart, TAS 7001, Australia
2 Centre for Crop Systems Analysis, Wageningen University, Netherlands
e-mail: holger.meinke@utas.edu.au
ABSTRACT
Change creates risks and opportunities. We can try to prevent it, resist it or adapt to it. The recent rate of environmental change has created urgency for well-planned adaptation action. It might be timely to consider the concept of ‘adaptation science’ as a process that generates the insights needed to affect changes in agricultural systems towards increased adaptive capacity and performance. This requires quantitative assessments of threats, risks and opportunities. It also requires appreciation and understanding of the socio-economic drivers of change. For adaptation science to have high social impact it needs to be salient, credible and legitimate.
Keywords: risk management, climate change, adaptation science, systems modeling
Introduction
We live in a world of increasingly rapid changes. Change creates risks and opportunities. Change is also a simple fact of life. Nature’s response to change is evolution. To successfully tackle the challenges of the 21st century – feeding over nine billion people sustainably - we need to ‘turbo-charge’ evolutionary processes; we need to become proactive in order to keep abreast of the continuously accelerating change process. We need to actively adapt.
When forces of change begin to exert pressure, there are only 4 possible responses:
Whatever we do – or whatever we don’t do – in response to this pressure is our choice; even inaction is a choice. And ultimately, we are all accountable for our choices. This means that we are inevitably part of the change process in a constant struggle to improve the human condition. We cannot escape it. Nobody can.
Agricultural science in all its complexity needs to play an active role in this rapidly changing world. Specifically, I want to highlight the importance of a systems analytical approach to ensure profitable and ecologically sound crop production. I want to highlight the relevance of such research for our societies. Many of my examples will refer to climate as a key driver of change in agricultural systems. Even though I will focus on climate risks, the approach I am suggesting is about risk management in agriculture generally, regardless of the underlying driver. However, given the importance of climate risks - and lately of course the increasing need to adapt to a changing climate – it is appropriate to focus on climate risks.
DISCUSSION
I have already mentioned the four possible responses to change: inaction, denial, mitigation and adaptation. When it comes to global and climate change, inaction and denial are no longer acceptable responses. By now we know far too much about the processes responsible for climate change; we also know far too much about their consequences to simply sit back and do nothing. It would be immoral to leave all the consequences of our affluent lifestyles simply to future generations to deal with. I fear that they will judge us harshly anyhow; and rightly so.
This leaves us with mitigation and adaptation. Now mitigation is most effective when action is taken early in order to prevent the system to evolve to a stage where drastic changes will occur. I think it is too late for this. Just to re-enforce this point, here is a very famous diagram, which I am sure you all have seen before in one form or another. It is a continuation of Keeling’s original atmospheric CO2 concentration curve (Fig. 1).
The beginning of Keeling’s CO2 measurements coincides with the year of my birth. The measurements show that in my lifetime alone, CO2 has increased by 85ppm, from 315ppm to nearly 400ppm today. Compared to the pre-industrial levels of around 280ppm, CO2 concentrations have now increased by 120ppm or 43%. We will be well into the 400ppm range by the time I will retire. Not too many statisticians would argue with me about this extrapolation. I regard this as a fairly certain; it is a foreseeable event.
There is something else that is close to certain: these increases in greenhouse gasses (GHG) are already impacting on our climate and climate sensitive sectors of our society. At a global scale we can see the impacts, many of them, from melting ice caps, retreating glaciers to the extinction of species, because there is no mountain high enough for them to escape to. We monitor and measure species diversity and we know that biodiversity is seriously under threat (for many reasons, not just climate change). But from here on, any certainty quickly dissipates.
Now let’s have a look at a second famous diagram. This is work by Rahmstorf from 2007 (Fig. 2). It shows two different measurements of annual, global temperature data with the IPCC projections for the different emission scenarios superimposed. I only want to point out one aspect of this diagram: it shows that ever since the IPCC began issuing projections, sometime in the mid 1990s, we have been tracking at the upper end of the scenario envelope.
And yes, I know, every single piece of scientific evidence is contentious, can be disputed. The point is: it is not the individual pieces of evidence that matter. What matters is that collectively the case is so compelling that inaction amounts to malicious negligence. Just a few very selective examples should suffice (Fig. 3):
Consider the rapidly melting ice caps and glaciers; remember the European heat wave of 2003; or the fact that 2012 was again amongst the 10 warmest years on record with sea ice extent in the Arctic reaching a record low. In fact, the Arctic continued to warm at about twice the rate compared with lower latitudes, while sea levels are at a record high, increasing at an average rate of 3.2 (+/- 0.4) mm (NOAA, 2013). And in January 2013, Hobart, Australia’s most southern capital city on an island surrounded by the Southern Ocean reached 43oC for the first time ever.
Or consider the Australian floods and bush fires of February 2009 that killed 200 people – these events occurred simultaneously, in different parts of the country. Fig. 4 shows the land surface temperature anomalies in the week leading up to these disasters; it clearly shows the intensity of these two contrasting extreme events.
Meanwhile many of our natural ecosystems such as coral reefs - places of incredible bio-diversity and beauty - are destroyed at alarming rates by increasing ocean temperatures and acidity. In short: the accumulated evidence is overwhelming.
Which brings me back to my earlier comments: Mitigation, that is the avoidance of processes that lead to climate change, is most effective and cheapest when taken early. This means that we should have begun decarbonising our economies way back in the 1960s when CO2 levels were still below 350ppm. We chose not to do this.
The choice not to act has two consequences: Firstly, it increases the urgency for mitigation action to avoid further substantial damage and secondly it increases the urgency to adapt as a certain amount of climate change is now unavoidable (Howden et al., 2007).
Although mitigation is politically extremely difficult, scientifically we can competently approach it: We need to reduce our GHG emissions by decarbonising our economies. This is a paradise for research industries and it creates much room for disciplinary-based innovations: from alternative energy sources to carbon capture such as clean coal technologies and underground CO2 storage facilities; reducing methane emissions from rice paddies or livestock (just think about the new breed of sheep that burp less) to riding your bike instead of using the car. While there is one goal, namely the reduction of GHG emissions, the means of achieving this goal are diverse and varied – the more, the better (Table 1).
Yet, politically mitigation is difficult; very difficult. In Australia we are in the middle of an election campaign where our very modest carbon tax is a very hot political issue. But if we think that mitigation is difficult, with adaptation, things get even more complicated as there is no single, agreed goal of what adaptation is supposed to achieve. The issue of competing claims and conflict agendas looms largely in the adaptation debate. Questions need to be answered such as: Who is adapting to what and with which consequences? And the issue of scale suddenly raises its head even more than before.
While reducing GHG emissions at any scale anywhere contributes to the ‘global good’, which is the overarching goal of mitigation, adaptation actions are largely independent, local and very context-specific. Hence, compared to mitigation, real adaptation actions are even harder to agree on and incredibly complex to achieve. Adaptation actions are highly contestable: what works well for certain sections of a community, can be to the detriment of others (Nelson et al., 2010).
Many people still object to the fact that something as dangerous as humanly induced climate change can actually result in opportunities and benefits for certain sectors and groups of individuals. This leaves science with a big conundrum: how can we best apply our narrow and very specific scientific knowledge to the issues of adaptation? How can we capitalise on opportunities without causing further damage?
Although adaptation is nothing new, the scientific adaptation agenda is – until only a few years ago, adaptation to climate change was seen by many as an issue to be avoided because it gave the impression of inevitability, of a system out of our control. It was regarded as politically dangerous and damaging. However, the evidence and urgency has now increased to such an extent that even most of the die-hards acknowledge that the time for adaptation action is now.
I want to briefly put adaptation in relation to agriculture in historical context. For this I will use 3 examples:
1) The Norse
Exactly 1027 years ago, in 986, Erik the Red led a group of Viking families from Iceland to settle in the southern parts of Greenland. 422 years later, in 1408, a wedding performed in the Hvalsey Church (Fig. 5) is the last recorded event before all settlements were again abandoned (Lundberg, 1999). What went wrong?
At the time of settlement, Greenland’s climate was considerably warmer than during the 20th century. The new settlers were farmers engaged in animal husbandry and cropping, who managed to accumulate enough food and fodder during the short summer season for a reasonable living.
However, the mild climatic conditions were fairly short-lived in geologic terms and by about 1200, progressively cooler conditions made life extremely difficult. In some years, no supply ships were able to reach Greenland due to persistent sea ice preventing the passage of ships (Lundberg, 1999). By the early 1300s, significant changes in temperature are recorded in such diverse materials as glacier ice, fossil vegetation and pollen deposited in lake sediments, chemical signatures in isotopic composition of sea sediments, animal and human bones (Fricke et al., 1995). This cooling climate led to agricultural practices that became progressively less sustainable and, as a consequence, depleted Greenland of its natural capital - its grasslands, animal resources and cropping opportunities.
For instance, fossil pollen indicates that the expansion of grasslands into increasingly marginal areas led to erosion. Land degradation forced people to push production even further until it exceeded the regenerative capacity of Greenland's environment, leading to a downward spiral of productivity and ultimately starvation (Panagiotakopulu and Buckland, 2007). Isotopic evidence from ice cores indicates that the 14th century was the coldest known in Greenland for 700 years.
Today, the arctic is warming at twice the rate of the rest of the world and agriculture is predicted to increase significantly in a region so far free of many pests and diseases (Jensen et al., 2009). Greenlanders are proud of the fact that they are again engaged in agriculture: home-grown potatoes, broccoli and strawberries have recently become part of the Greenland diet, while options for growing barely on a larger scale are being explored (Fig. 6). Greenland is capitalising on some of the arising opportunities from climate change.
2) The Potato Eaters
Let me move on to another example: This evocative painting by Vincent van Gogh (Fig. 7) is called ‘The Potato Eaters’. Vincent painted it in 1882 and it is a classical example of human adaptive capacity. Very skillfully it symbolises multilevel adaptation: while the problem was largely climate induced poverty and famine – caused by natural climate variability – one solution was the growing and eating of potatoes.
Potatoes were first introduced into Europe in the mid 16th century, just at the beginning of the ‘little ice age’ when crop yields generally were declining. The key innovation was that potatoes provided 2-3 times more food per unit land compared to cereals such as wheat. The introduction of potatoes into Estonia in the 1840s put an end to frequently recurring famines that had killed over 75,000 people in Estonia alone (Tannberg et al., 2000).
By the mid 19th century potatoes were therefore considered the ‘bread of the poor’, a staple food and a reliable substitute for wheat. So out of necessity, poor European farmers adapted their cropping systems as well as their eating habits; adaptation is always multi-faceted.
This is risk management in action – risk management defined as the systematic process of identifying, analysing and responding to risk. It includes maximising the probability and consequences of positive events and minimising those of adverse events. Good risk management seizes opportunities without ideological biases.
The recent pace of global change has added an additional component of urgency for planned risk management and proactive adaptation. It is now time to address this issue more systematically, particularly when it comes to agriculture.
This brings me to the emerging field of ‘adaptation science’ (Meinke et al., 2009): Adaptation science is not a new science – it is simply a different way of conducting science. The traditional, reductionist approach to science has a tendency to create islands of knowledge in a sea of ignorance. Traditional science focuses much more on analysis of scientific inputs rather than on the synthesis of socially relevant outcomes.
Adaptation science, on the other hand, is very much outcome, solution oriented. This new way of scientific inquiry differs from the traditional approach of ‘science for adaptation’ by attempting to provide societal relevant solutions rather than just more disciplinary based data. Adaptation science is a problem focused and solution oriented science paradigm that, without prejudice, tries to combine societal relevance with scientific excellence. It has a lot in common with the notion of ‘adaptive management’, an ecological approach to action increasingly popular within the conservation movement. But more of this later.
Given the urgency created by the accelerating pace of climate change, climate risks serve as a good example of how adaptation science might bring together scientific knowledge from many disciplines in order to manage our limited resources more sustainably and profitably. And although we cannot predict with certainty what is going to happen, many of the problems that are in-store for us are very well foreseeable.
The concept of foreseeability is key here: the best example I can think of that distinguishes foreseeable events from predictions is when you run a red traffic light at a busy intersection. I cannot predict that you will have an accident, but I can foresee that your chances of having an accident will be dramatically increased. Foreseeability is a legal concept that dictates that if a disaster or an accident is foreseeable, but the operator of the system does not take necessary precautionary action he or she will be liable. Future generations will hold us to it.
Adaptation science takes such risk management principles seriously. Science today offers us the unique opportunity to (a) better understand causes and consequences of our actions and (b) learn from history, by being better prepared and willing to adapt.
Let me give you a final historical example to underline the need to adapt, this time from my home continent, Australia:
3) South Australian settlers in the late 19th century
South Australia’s ‘Goyder Line’ is an icon in Australia’s agricultural history. The line was first drawn by George Goyder, a geographer extraordinaire and South Australia’s surveyor-general at the time.
Driven by a deep, intuitive understanding of the role of rainfall variability as a key driver of risk for wheat farming in low rainfall regions, Goyder set out in 1865 to determine 'the line of demarcation between the portion of the country where the rainfall has extended and that where the drought prevails' (Government of South Australian, 2010).
George Goyder went out on a horse, examined the natural system, particularly plants and soils, and drew inferences from this knowledge. Based on this, he drew a line on a map to highlight the areas where, in his opinion, cropping should never be attempted (Fig. 8). The line runs roughly west to east across the mid-north of SA from the edge of the desert, the Nullarbor Plain, to the Victorian border (between 33o and 34o S; ABC, 2009).
Goyder was a visionary and one of the very first people to perceive the degree and impact of Australia’s highly variable rainfall. He postulated that north of his line, which equates roughly to the 300mm annual rainfall zone, rainfall was too low and erratic for viable wheat production. These days we use simulation models to arrive at such conclusions. Goyder conducted this systems analysis in his head!
Just a few years later, however, Goyder’s line became highly controversial when a series of wet years resulted in farmers establishing properties way north of his line. He was attacked in parliament, ridiculed in the press. At public meetings, people called for Goyder and his line to be thrown out of the colony (ABC, 2009). When more variable and drier conditions returned yet again in the late 1880s nearly all of the new farms were abandoned resulting in considerable hardship.
Goyder’s line had an enormous influence on the Australian psyche and rural settlement policies. He was at least one century ahead of his time in understanding rainfall variability and its impact on natural ecosystems and agricultural productivity. Since then generations of Australian farmers have learned at their peril to ignore climate variability and its consequences on farm businesses. As a consequence, Australia has probably one of the most ‘climate aware’ agricultural sectors in the world.
Lessons learned?
The experience of the Norse, the early Australian settlers and poor European farmers should be sufficiently convincing that adaptation science – a more formal, transdisciplinary approach to scientific inquiry – is urgently needed. But how will it work? What do we need to do differently when compared to what we already do?
In the examples I provided, everyone would have benefited from the knowledge-generating technologies that are at our disposal today. For agriculture ‘creating hindsight in advance’ has only become possible based on the scientific breakthroughs in modelling complex systems. This modelling work (i.e. ‘system thinking’) has its origin at Wageningen University in The Netherlands, where Prof. Cees de Wit first introduced systems theory and simulation modelling in the 1950s and 60s. The global science community continues to build on the foundations de Wit created.
Today we use models to create a new type of knowledge that is similar to the conventional, empirical knowledge that decision makers usually draw on when managing risks holistically and through intuition (e.g. Keating et al., 2003; Meinke et al. 2009). This provides the cornerstones for adaptation science, which draws heavily on such knowledge-generating technologies. The ability to analyse and simulate complex systems is essential for ‘creating hindsight in advance’.
I already outlined the need for adaptation science; I will now argue that this way of doing science is highly context-specific and needs to be in itself ‘adaptive’. This might even include a requirement for new, different institutions and the way they interact.
So how will adaptation science work? For this I propose a 4-step approach (Fig. 9; Meinke et al., 2009):
Let us for now assume that we have established such a community of practice around the issue of climate risk in agriculture. One of the first hurdles we have to overcome, even before we turn this into a truly transdisciplinary community, is breaking down the barriers within disciplines. While much of the talk is about climate change, the real issue from a farmer’s perspective is managing scarce resources such as land, water and labour within the context of a risky environment. What we often forget is that climate change will be delivered to the farm gate – free of charge – by climate variability (Meinke and Stone, 2005). As one of my colleagues often quips: ‘Who destroyed the sand castle? Was it the wave or was it the tide?’ (Fig. 10). This is a powerful analogy that stresses how existing climate variability rides on a slowly rising tide. Unless we help the rural sectors in both developing AND developed countries to proactively adapt to climate variability and change, we fail in our responsibility as scientists.
There is no doubt that plants, and hence crops, will remain the single most important objects in the quest to solve the problems of our planet. Without plants there will be no life. A systems analytical approach is uniquely placed to provide the underpinning science for adaptation actions. We can now develop and apply the quantitative modelling tools that are needed to assess the value of different adaptation options for agricultural production systems.
Good crop modelling at the scientific forefront of understanding how plants and plant systems function. To better understand and assess the function of these systems, we use simulation modelling as one of our core technologies. Ideally a good modelling team should be multi-disciplinary including scientists comprising plant physiologists, crop physiologists, cropping systems experts, ecologists, modellers and climate scientists – to name just some of the key disciplines that are essential for problem solving in agriculture.
Of course, in spite of being a multidisciplinary team, often additional expertise is needed to solve real life problems. Hence, global collaboration is the key to a group’s success. Collaborative networks should be extensive and reach all corners of the earth. This is one of the reasons why I am here today.
Let me give you an example that might resonate with you:
Rice is the world’s most important staple food; 91% of all rice is produced in Asia. There is limited scope to increase production due to competing claims on scarce resources such as water, land and labour. Increasing temperatures, more frequent droughts, anticipated loss of productive estuaries caused by rising sea levels and more frequent and severe storms further compounds these problems. This creates urgency for rice-based production systems to produce more rice with less water, less land or less labour; in other words: we need to increase these various efficiencies, which are also referred to as ‘eco-efficiencies’.
In his classic paper on “Resource Use Efficiency in Agriculture”, Cees de Wit (1992) explored these eco-efficiencies. His paper sparked intense scientific debate, which, unfortunately, I don’t have time to detail here. There is agreement, however, that eco-efficiencies are highly contextual. To understand these complexities, we need formal, quantitative methods that help us to decide, which efficiency improvement to target for maximum gain. For this, an efficiency frontier framework can provide valuable guidance when making research investment decisions (Fig. 11; Keating et al., 2010):
The diagrams are stylised presentations of input / output relations for agricultural production. They describe general responses to inputs at various levels of intensity and co-limitations. Particularly under severely limited conditions, this approach can help to determine the most appropriate starting point in order to increase productivity and efficiency of production. How do we know which type of technology might be best suited for such improvements? To answer this question, we consider 3 different levels of input / output relationships in a developing country context:
Let us explore how we can use this framework operationally:
Increasing inputs can increase outputs, but it usually also increases output variability, particularly when climate is highly variable. Therefore, the variance in economic returns can be used as a surrogate measure for risk. On the x-axis of Fig. 11b we replaced inputs with a measure of risk, namely the variance in economic returns of a certain action. The y-axis shows the longterm or mean economic return of a certain action. I will now briefly demonstrate how such efficiency frontiers can be used as risk management tools:
With the help of models, the necessary data for such response functions can be derived and foreseeable events can be probabilistically described (Maia and Meinke, 2010) and then used for a range of stakeholders who can then engage in meaningful dialogues amongst themselves (Fig. 12).
Once this is achieved, the framework can be used to diagnose the most promising pathways for intervention into agricultural systems to improve their performance – either incrementally or via fundamental changes to the system. Used in this way, integrated adaptation science can really help to improve the livelihoods of the most vulnerable people.
So to improve the productivity of rice-based systems, we need to explore all options, incremental improvements and revolutionary change. What works best will depend on the context. We need incremental improvements, for instance better water management based on seasonal climate forecasts, improved infrastructure and adapted varieties. This moves us along or onto the existing efficiency frontier. We also need the transformational changes in places where there is simply not enough irrigation water left.
For rice-based systems in water-scarce environments, this is when aerobic rice – that is rice that is not grown under flooded conditions – comes into play (Fig. 13). While production per unit area will not increase or possibly even slightly decrease under aerobic conditions, water use efficiency gains of more than 100% are clearly achievable. In terms of water use, this will move us onto a new efficiency frontier.
This is ‘adaptation by design’ – a careful analysis of all the options before implementing the most promising ones. Clearly, this requires good modelling skills at all scales that draw strongly on expertise across an extensive network of scientists.
I would rather like to briefly touch on three more points:
Firstly, the importance of teaching and education
The success of a university depends very much on a strong commitment to diversity and true inclusiveness. We must the education of the next generation as our civic duty rather than a privilege that is only accessible to some. The importance of equity and inclusiveness cannot be overrated. This has consequences for our institutions, particularly our universities:
The second point I would like to stress is the importance of flexibility, tolerance and self-reflection
We can’t talk about adaptation without adapting ourselves. In fact, this is one of the principles of adaptive governance of which adaptation science is a logical extension. In my group, rather than fixing our structure and our strategic direction, we constantly reflect and review. In a world where the pace of change is rapidly increasing, we must remain responsive to these changing demands. True to the principle of life-long learning, we endeavour to be a responsive and proactive group that constantly re-invents itself rather than defending our past. Following the principles put forward by Hamel and Välikangas (2003), we aim to achieve revolutionary change in lightning-quick, evolutionary steps, but with no calamitous surprises, no convulsive re-organisations, no across-the-board staff changes. In a truly resilient organisation, there should be plenty of excitement, but no trauma. At the very least we should try creating work environments where great ideas and innovation can thrive, but where the direction is clear and shared by all.
And finally: social change
Agriculture has been the engine for social change in human history. Without an abundance of food, there wouldn’t be any change. So isn’t it ironic that rural people and regions are now perceived as the ones most resistant to changes? Maybe having lost control over the forces agriculture created, there is a now tendency to ‘dig in’, circle the wagons. Although this is an understandable reaction, it is not a strategy that will succeed. The global forces are far greater than any resistance can withstand. But there are alternatives:
The 1960s and ‘70s were the decades of the green revolution, based on research-based, technological breakthroughs with all their positive and negative consequences. This was followed by a period of relative calm. Now, with climate change and the emerging pressure on our resource base and rapidly growing populations on our doorstep, we have entered into another type of agricultural revolution: the need to feed a growing population sustainably without increasing our resource use.
Being a serious player in this new round of transformational changes requires knowledge and wisdom. These are commodities that are not sold in 20kg bags. Knowledge is hard to acquire and it takes time and effort. The people who will influence the outcomes of this new revolution will be well-educated, and not just in agricultural practice. Yes, they will understand the principles of farming, but they will also be experts in global finances; they will understand the importance of sustainable practices; they will be effective mangers of human resources and they will shape the inevitable debates about contentious issues. They will combine business acumen with social intelligence. In short: they will be real leaders. And we, the agricultural research community, need to be among them, driving this change.
CONCLUSION
The pace of environmental and social change will accelerate. Social changes will be led by groups and individuals who are highly educated and influential. The best we can hope for is that as many of these change agents as possible will have a solid understanding of agricultural systems and their role in our societies. We need to get amongst them.
Agriculture and Food Systems ARE the new black! Universities have a responsibility to provide the rounded education that the current and future players in this knowledge-intensive game need to succeed. We are in the midst of a knowledge-based revolution and we need to take charge of it. We need to ensure that the next generation of scientific leaders understands agriculture. Tertiary education has never been as important as right now, so now it not the time to pull back and divest from the tertiary sector. ‘Creating an abundance’ of knowledge and wisdom is the best investment we can make. We need productive minds to manage our productive landscapes. We need a productive tertiary sector.
REFERENCES
ABC (Australian Broadcasting Corporation), 2009. http://www.abc.net.au/rn/hindsight/stories/2009/2540163.htm
Australian Bureau of Meteorology, 2010. Annual Australian Climate Statement 2010. http://www.bom.gov.au/announcements/media_releases/climate/change/20100105.shtm
Carlson, M., 2006. History of Medieval Greenland; http://www.personal.utulsa.edu/~marc-carlson/history/grontimebible.html
Fricke, H.C., O'Neil, J.R. and Lynnerup, N. 1995. Oxygen isotope composition of human tooth enamel from medieval Greenland: Linking climate and society. Geology 23, 869-872.
Government of South Australian, 2010. State records of South Australia. http://www.archives.sa.gov.au/exhibits/saonmap/gwgtimeline.html
Hamel, G. and Välikangas, L. 2003. The Quest for Resilience, September 2003, 1-13.
Howden, S.M., Soussana, J-F., Tubiello, F.N., Chhetri, N., Dunlop, M. and Meinke, H., 2007. Adapting agriculture to climate change. PNAS, 104(5), 19691–19696. doi:10.1073/pnas.0701890104.
Jensen, C., Munk, L., de Neergaard, E., Høegh, K. and Stougaard, P., 2009. Biocontrol bacteria isolated from potato fields in Greenland. Climate Change: Global Risks, Challenges and Decisions. IOP Publishing IOP Conf. Series, Earth and Environmental Science 6; doi:10.1088/1755-1307/6/7/372041.
Keating, B.A., Carberry, P.S., Hammer, G.L., Probert, M.E., Robertson, M.J., Holzworth, D., Huth, N.I., Hargreaves, J.N.G., Meinke, H., Hochman, Z., McLean, G., Verburg, K., Snow, V., Dimes, J.P., Silburn, M., Wang, E., Brown, S., Bristow, K.L., Asseng, S., Chapman, S., McCown, R.L., Freebairn, D.M. and Smith, C.J., 2003. An overview of APSIM, a model designed for farming systems simulation. European Journal of Agronomy, 18: 267-288.
Keating, B.A., Carberry, P.S., Bindraban, P., Asseng, S., Meinke, H. and Dixon, J., 2010. Eco-efficient agriculture: concepts, challenges and opportunities. Crop Science, accepted.
KNMI (Koninklijk Nederlands Meteorologisch Instituut), 2010. http://www.knmi.nl/klimatologie/maand_en_seizoensoverzichten/index.html
Lundberg, M., 1999. The history of Greenland. http://explorenorth.com/library/weekly/aa121799.htm
Maia, A.H.N. and Meinke, H., 2010. Probabilistic methods for seasonal forecasting in a changing climate: Cox-type regression models. Int. J. Climatology., in press, doi:10.1002/joc.2042.
Meinke, H., 2010. Adaptation Science for agriculture - solutions for a changing planet. Inaugural lecture, Wageningen University, 14 January 2010, ISBN 987-90-8585-271-1, pp. 34.
Meinke, H. and Stone, R.C., 2005. Seasonal and inter-annual climate forecasting: the new tool for increasing preparedness to climate variability and change in agricultural planning and operations. Climatic Change, 70, 221-253.
Meinke, H., Howden, S.M., Struik, P.C., Nelson, R., Rodriguez, D. and Chapman, S.C., 2009. Adaptation science for agricultural and natural resource management – Urgency and theoretical basis. Current Opinion in Environmental Sustainability, 1, 69–76. doi:10.1016/j.cosust.2009.07.007.
Nelson, R., Kokic, P., Crimp, S, Meinke, H. and Howden, S.M., 2010. The vulnerability of Australian rural communities to climate variability and change: Part I – Conceptualising and measuring vulnerability. Environmental Science and Policy. 13, 8-17. doi:10.1016/j.envsci.2009.09.006.
NOAA, 2013. State of the Climate in 2012: Highlights. http://www.climate.gov/news-features/understanding-climate/state-climate-2012-highlights
Panagiotakopulu, E. and Buckland, P., 2007. Fossil insect evidence for the end of the Western Settlement in Norse Greenland. Naturwissenschaften 94, 300–306; DOI 10.1007/s00114-006-0199-6
Rahmstorf, S., Cazenave, A., Church, J.A., Hansen, J.E., Keeling, R.F., Parker, D.E. and Somerville, R.C.J., 2007. Recent climate observations compared to projections. Science 316, 709.
Tannberg, T., Mäesalu, A., Lukas, T., Laur, M. and Pajur, A. 2000. History of Estonia. Avita, Tallinn, 335 p.
Wit, C.T. de 1992. Resource use efficiency in agriculture. Agric. Systems, 40, 125-151.
[1] * This talk is largely based on my inaugural lecture (Meinke, 2010)