ABSTRACT
Biogas, which is comprised of methane and carbon dioxide, is a renewable energy source that is produced through anaerobic digestion by a consortium of anaerobic microorganisms. Various organic materials, including organic wastes, organic fractions of municipal solid wastes, wastewaters from agro-industries, and energy crops, can be utilized as a substrate for biogas production. Biogas helps a community or a country to move towards energy security, since it can be used to replace heat or to generate electricity. Many developed countries, such as Germany, have successfully implemented an integrated renewable energy platform that includes biogas and other renewable energy resources, e.g. wind and solar, both for environmental benefits and economic revenues. Electricity from renewable energy sources has become a commodity. On another note, renewable energy markets have not been widely achieved in developing countries. Most countries still focus on waste treatment and utilization due to several barriers. In some cases, government policy is the main barrier to the promotion of renewable energy usage. This paper reviews the current status, promotion policy, and limiting barriers obstructing the opportunity for biogas utilization as a commodity. We hope that in the near future biogas, as a renewable energy source and as an originated component for other value-added products, will become fully exploited.
Keywords: Biogas, energy security, environmental benefit, waste treatment, renewable energy
INTRODUCTION
Thailand has relied on high levels of imported energy, at approximately 50% of all its energy consumption and increasing since the mid-1980s (Environment, 2014). In 2020, the total energy consumption in Thailand was equal to 76,928 ktoe, of which 15.1% was derived from renewable energy (RE) (D. o. A. E. D. a. Efficiency, 2021). The major RE consumption is in the form of heat, but the share of electricity and biofuels as RE is growing. The Alternative Energy Development Plan was first implemented in 2012 with the aim of increasing the proportion of alternative energy consumption to up to 30% of the total energy consumption (adjusted in 2019). Further, Thailand’s 20-year National Policy Plan (2017–2036) focuses on bio-economy, circular economy, and green economy (BCG Economy). This concept can solve natural resource problems and improve the environment and social sustainability. The BCG economy for Thailand will focus on using raw materials more efficiently, minimizing waste, improving production efficiency, upgrading technology, and creating innovation for higher efficiency. Biogas, a renewable energy source, can be one BCG solution for the renewable energy promotion scheme as well as an alternative for reducing waste and environmental impacts, subsequently reducing greenhouse gas (GHG) emissions (N. S. a. T. D. Agency, 2018).
Biogas is a naturally-occurring biological process, in which anaerobic microorganisms transform organic matters to methane (CH4) and carbon dioxide (CO2) in the absence of oxygen. The advantages of an anaerobic process for organic waste treatment are that it requires less energy and less space for building a treatment system than that of an aerobic process. Biogas systems have been utilized as waste and wastewater treatment units in various sectors: agriculture, agro- and food industries, and even the energy-producing sector. Raw materials for biogas generation are agricultural residues, wastes from animal farms, wastewater from agro- and food industries, or the organic fraction of municipal solid wastes.
A typical biogas system has three main components: raw material preliminary unit, biogas production unit, and post-treatment units (Figure 1). The objectives of the preliminary or pre-treatment unit are raw material size reduction, removal of inorganic materials, and toxicity neutralization. Pretreatment technologies are mechanical, thermal, enzymatic, and chemical treatments.
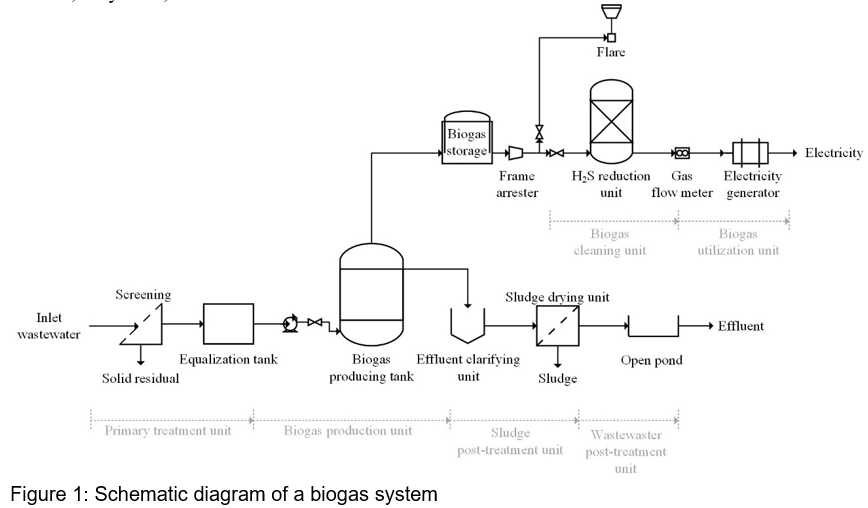
A biogas reactor, or biogas producing unit, can be classified in several ways, based on the system efficiency, the microbial structure inside the reactor, the operating temperature, or the amount of solids entering the system. The biogas system based on the efficiency can be divided into low-rate, conventional rate and high-rate systems, which are classified by the organic loading rate of the system (<2, 2-4, >4 kg of COD/m3 of reactor/day, respectively), where COD stands for chemical oxygen demand, indicating the amount of organic matter. There are two types of microbial structure inside the reactor: suspended growth and attached growth. The reactor with the suspended growth type is where anaerobic microbial consortium forms anaerobic granules suspending inside the reactor, while, with the attached growth type, the anaerobic microbial consortium forms a biofilm, which attaches onto the media inside the reactor. The two operating temperatures are mesophilic (approximately 35oC) and thermophilic (approximately 55oC). The solids entering the reactor influence the design of the system. A typical reactor that has the influent of <20% of the solid content called a wet system, while a reactor with >20% of solid content is called a dry system. Biogas technology is at a fully commercialized level (Suwanasri et al., 2015). Most biogas systems require post-treatment for all three phases of the effluent – secondary treatment open ponds for the wastewater, biogas cleaning for the biogas, and sludge treatment or utilization for the solid waste. Most biogas cleaning technologies to remove contaminants, such as hydrogen sulfide (H2S), are at fully commercialized levels. In general, the utilization of biogas in Thailand is in the form of heat, electricity, vehicular fuel, and combined heat and power, and all of which are already in fully commercialized levels (Suwanasri et al., 2015).
BIOGAS AS WASTE TREATMENT
Implementation of the biogas system for waste and wastewater treatment options has been initiated in Thailand since the early `80s. The biogas system was first promoted in animal farms as a waste treatment unit. Unfortunately, it was unsuccessful due to a lack of understanding and construction standards leading to the system failure. To overcome these drawbacks, the Thai government created initiatives in collaboration with the German Technical Corporation, which is now known as the German International Corporation for the promotion of the biogas systems in animal farms. Universities collaborated with the Department of Agricultural Extension, Ministry of Agriculture, to disseminate knowledge on the Thai biogas technology for the treatment of wastewater from animal farms. Further, in the 2000s, the Ministry of Energy promoted the use of biogas systems in agro-industries utilizing local technology from universities and private companies.
One success case of biogas technology transfer in Thailand is the transfer of high-rate anaerobic fixed film (AFF) technology from King Mongkut’s University of Technology Thonburi and the National Center for Genetic Engineering and Biotechnology to agro- and food industries, especially cassava starch, palm oil, and food factories (shown in Figure 2). Media installation in the AFF helps preventing sludge wash-out, as shown in Figure 3(a). The AFF has high microorganism concentration, consequently high system efficiency and resistant to high-concentration wastewater. Each biogas system was designed based upon the type of wastes and wastewaters, the amount of waste fed into the system, and the selected technology. Factories selected the biogas utilization option based upon and customized to their needs, receiving economic benefit along with fulfilling their waste treatment obligation. Table 1 presents the biogas utilization alternatives with an estimated economic impact in each type of industry using this high-rate technology.
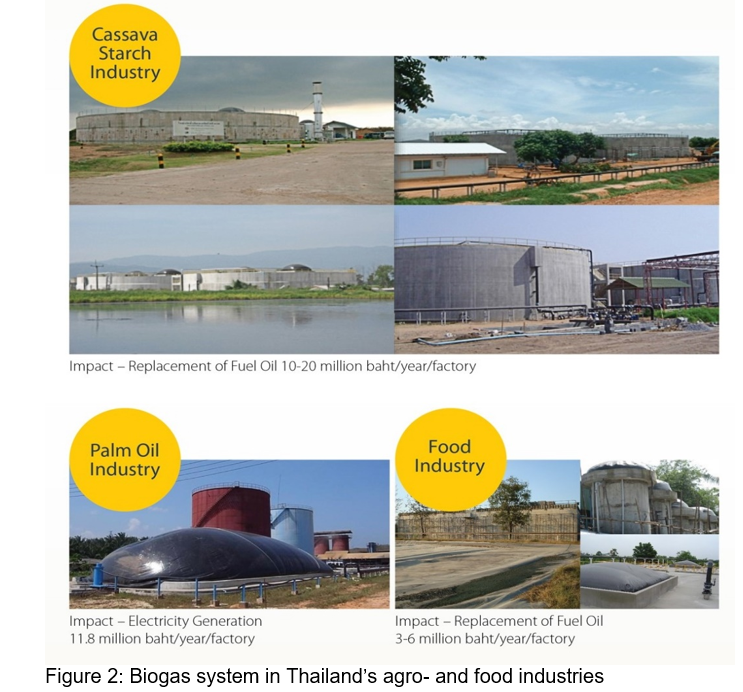
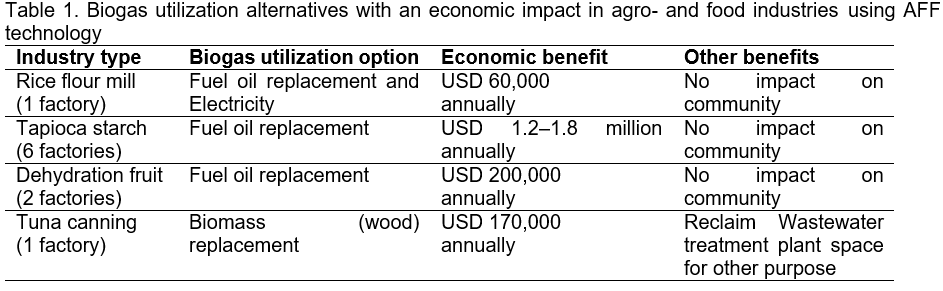
Another biogas technology adoption can be seen at the Charoen Pokphand Foods (CPF). The CPF implemented the conventional-rate anaerobic baffled reactor (ABR) technology for treating wastewater from animal farms. Baffles inside the ABR control direction of fluid flow, make it possible to extend the sludge retention time, as shown in Figure 3(b). The project costs approximately USD 800,000, which the Energy Policy and Planning Office, Ministry of Energy, partially subsidized (Buranasingh, 2020). The ABR helped eliminate the smell of the poultry manure, which was of concern to the nearby community. On the other hand, it generated biogas at 2,500 m3/d, which was used for producing heat and electricity, replacing 360,000 L/y of fuel oil. In 2019, the CPF and its affiliated companies produced 48.61 million-m3 of biogas, which saved a production cost of USD 7.8 million and reduced the GHG emission by 33,268 ton-CO2eq (C.P, 2020).
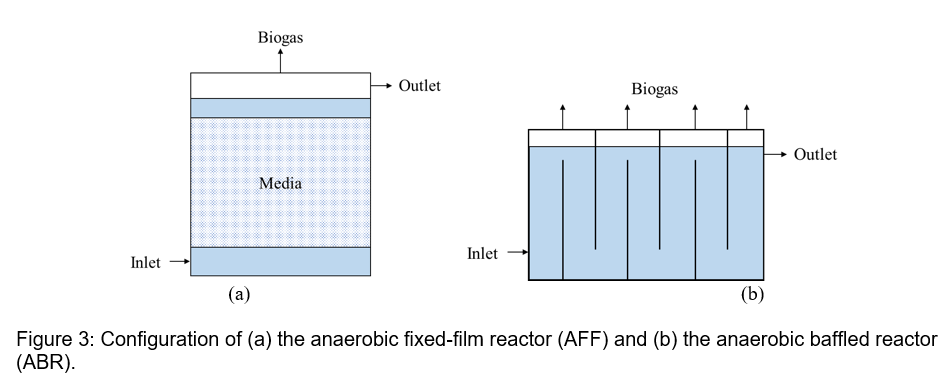
The other success case of the biogas implementation in the cassava starch industry was at the Korat waste to energy project (KWTE), in 2003. The project set up a business model between a biogas company and a cassava starch factory, as the Built-Own-Operate-Transfer (BOOT). The biogas company invested in biogas production along with electricity production, while the cassava starch factory provided land, wastewater, and the commitment to buy the electricity from the biogas company. Under the BOOT agreement, the electricity price was set to be 20% lower than the grid. In the first year of operation, the biogas plant supplied the energy replacing the 7.5 million L of fuel oil and 35 GWh of grid’s electricity, which was almost self-sufficient for the cassava starch factory. The KWTE overperformed with a high profitability, at the IRR of 15–17%, along with promoting an environmentally-friendly image to the cassava starch factory (Siteur, 2012). This success case attracted other companies to adopt the biogas production technology. Between 2004 and 2011, biogas technology installation in the Thai-cassava starch factories increased significantly. At the end of 2011, almost 80% of 77 Thai-cassava starch factories had installed biogas plants.
According to the calculations of the model for the production of biogas from cassava starch waste within ten years, the achievement of commercial benefits demonstrates a promising nature for investment in such enterprises by state corporations and financing through state programs to support climate and energy policy. Cellulosic material is a high potential raw material for the 2nd generation biogas production, in Thailand. Thai cassava starch industry also generates cassava pulp, as a solid residue of cassava root remained after starch extraction, of 12.5 million tons annually (Trakulvichean et al., 2017). With the circularity concept, several approaches have been attempted to utilize the cassava pulp for both agriculture- (animal feed, mushroom growth media, fertilizer) and renewable energy-sector (bioethanol, biogas, and refused derived fuel (RDF)). Producing the electricity from the cassava pulp through the biogas production technology is economically feasible (Lerdlattaporn et al., 2021). The investment on such technology depends on biogas utilization option, which is so far aimed mainly as heat source for the starch production process for producing the electricity. While the latter option is limited by the capability of the grid to buy-back the electricity and still needing the policy support on the electricity pricing mechanism. By-products from the cassava starch production are utilized under the circular economy concept. All end products, e.g., treated wastewater, sand, soil, peel, and cassava pulp can be used for the cassava root cultivation. So far, several factories import the technology but there are still lack of knowledge to utilize the technology. Which mislead technology feasibility to be questioned among the industries. These pulp utilization options need to be balanced with selling the pulp as animal feed to maximize the economic benefits for private companies. Then, the renewable energy production from the cassava pulp is needing the determination of the priorities for new cassava pulp markets, supportive policies, and a roadmap for achieving these priorities are also needed. Government initiatives may motivate cassava starch factories to choose beneficial options for the sustainable waste management of pulp. Biogas production from cassava pulp is still in need of government support to demonstrate its local proven biogas technologies are similar to biogas production from cassava starch wastewater (Trakulvichean et al., 2019).
POLICY ON BIOGAS PROMOTION IN THAILAND: PAST TO CURRENT STATE
In the `70s, the Sanitary Division under the Ministry of Public Health of Thailand began to invest in small-sized biogas plants for resolving a sanitary issue. As aforementioned, in the late 1980s, the oil price increased drastically worldwide, and so the Royal Thai government started to push biogas as an alternative energy source, which caused the major movement in developing an incentive policy for biogas plant investment (Intarangsi & Kiatpakdee, 2000). Initially, the investment cost in biogas, though effective productivity-wise, was too high to be attractive for the private sector (Suwanasri et al., 2015); therefore, the early incentive policy focused on increasing the financial competitiveness of biogas as an alternative power source. This was achieved through the measure of electricity pricing adder in the feed-in tariff system, the financial support from the energy conservation promotion fund, the investment promotion by the Thailand Board of Investment, and the promotion measure of technology on biogas production for livestock and other relevant enterprises. Different promotion schemes were locally implemented and were focused on both the promotion of the governmental sector and the private consulting firms for biogas production. For example, some projects have been implemented from those private consultants performed by the Thai Energy and Environment Development company and the projects from the governmental sector were supported by the Department of Alternative Energy Development and Efficiency (company, 2021; D. o. A. E. D. a. Efficiency, 2021b). From 2012, the national energy policy and the Alternative Energy Development Plan were implemented to increase the proportion of alternative energy consumption up to 30% of the total energy consumption, which became the second wave of the promotional policy for biogas.
The big difference in the recent incentive program was that the recent policy aimed to target the waste-to-energy perspective of biogas, especially municipal solid waste treatment, more than just alternative energy due to the enormous amount of waste produced in Thailand (Boonpa & Sharp, 2017). According to the rise of the circular economy, the future of biogas in Thailand might have to readjust its position by strengthening the environmentally friendly factors. According to the EU net-zero emission goals in 2050, the draft of the European Green Deal has been pushed to take a major effect in the supply chain of the EU in the commodities products, especially food sectors. Carbon Tax Adjustment and Tax Credit will be applied in order to justify the cost to go greener in food production, which causes producers who want to sell their products at a compatible price in the EU to seek greener energy sources to lower their carbon footprint (Krenek, 2020). In Thailand, from 2023 the Securities and Exchange Commission will highlight the awareness of alternative energy usage of a company by forcing the company to report its current GHG emission, current carbon footprint, and roadmap to go greener in the report for the annual general meeting of shareholders (SEC, 2021). These top-down enforcers will help push the members of the private sector in Thailand to make a higher proportion of their energy consumption come from alternative renewable energy sources, in which biogas has approximately half of the stake in Thailand (I. R. E. Agency, 2017; Office, 2020).
BIOGAS AS A COMMODITY IN THE GLOBAL ENERGY MARKET
The lack of affordable energy services is closely linked to many sustainable development issues, such as poverty reduction, the advancement of women, environmental protection, and job creation. The emphasis on institution-building and the expansion of political dialogue is necessary to create a social, economic, and political enabling environment to transition to a more sustainable future. On the other hand, technologies for generating energy from biomass are a promising option that will significantly impact developing countries where the current level of energy services is low (Omer, 2005).
Biogas has many advantages and is used for various purposes, such as electricity production, thermal energy production, and use as a transport fuel. Many countries have chosen the use of renewable energy sources as one of the priorities of their national policy, including the EU countries, the USA, Brazil, Canada, Japan, China, and others. In Europe, Germany is the leader in terms of the amount of biogas produced, while Denmark is the leader in terms of the intensity of biogas usage (this type of fuel provides almost 20% of the country's energy consumption). The policy elevated the number of biogas plants using energy crops as well as wastes from animal farms and factories to generate electricity, which can be sold as a commodity in an energy market. Further, within the Green Course program framework, the European Commission sets the task of building a climate-neutral European economy by 2050. One of the primary means of achieving it is the complete replacement of natural gas with carbon-free and renewable analogs: biogas, biomethanol, and hydrogen (H2).
The United Nations (UN) Commission on Sustainable Development (CSD) identified access to basic energy services as an essential element of sustainable development. According to the CSD, to implement the goal accepted by the international community to halve the proportion of people living on less than USD 1 per day by 2015, access to affordable energy services is a prerequisite (ITDG, 2007). There is a strong correlation between energy availability and education, health, urban migration, empowerment, local employment and income generation, and the overall quality of life.
The change in the global energy supply requires new solutions in the agro- and food industries, where biogas can become one of the drivers of economic growth in Southeast Asia. When making strategic decisions on creating enterprises for the production of biogas, it is important to take into account the economic benefits and the impact on the environment, socio-economic, and budgetary efficiency. The economic efficiency of biogas plants is especially high when processing a large continuous stream of wastes. In addition, biogas energy is also a source of cheap complex organic fertilizers, which are formed as a by-product in biogas production. At the same time, it is advisable to consider the production of biogas as the final link in the production chain for the disposal of production waste and the transformation of waste into a commodity with high added value. From the point of view of the producers of export goods from agro- and food raw materials, the possibility of creating a private-public partnership will allow the enterprise to monetize the costs associated with waste losses. From the state's point of view, an increase in revenues to the state budget is obtained by increasing the profits from export production and obtaining a share of electricity that can close or reduce the required level of electricity imports or to reduce the price of electricity due to income from biogas.
BIOGAS AS ORIGINATED COMPOUND FOR VALUE-ADDED PRODUCTS
Direct use of biogas (CH4 as natural gas, CO2 as gas or liquid use in greenhouse or industry)
By volume, biogas contains 50–70% of CH4, 30–50% of CO2, and small amounts of H2 and other contaminants, such water, siloxanes, volatile organic compounds, and H2S (Awe et al., 2017; Paolini et al., 2018; Tonrangklang et al., 2017). The proportion of CH4 in the biogas affects the heating value of the biogas, whereas CO2 does not provide heat (Yousef et al., 2016). A normal cubic meter of CH4 contains 10 kWh of energy (Yentekakis & Goula, 2017), which is close to that of natural gas (energy content of 11 kWh). Over the past decade, biogas has been upgraded to biomethane for injecting into the natural gas grid and even as compressed biomethane gas (CBG) as a fuel in the transportation sector (Efficiency, 2016).
In Thailand, the first pilot plant producing CBG, with a production capacity of 8 kg/h, occurred in 2012 (Wang et al., 2021). Permission and quality guarantee of CH4 gas are regulated before injection into the grid (Kadam & Panwar, 2017). In recent years, worldwide biogas utilization has not only focused on fuel but also its use as a raw material for biochemicals production (Hwang et al., 2018; Wang et al., 2021). Using biogas purification technologies, such as pressure swing absorption, CO2 is separated and can be delivered to various destinations. In the Netherlands and Belgium, CO2 is delivered to greenhouses for crop and flower production or it can be directly supplied to algae production, which is an energy crop and functional food. Trends of biogas utilization in Thailand are shifting in the same manner.
Transformation of biogas to other products: CH4, CO2, and H2
Purification of the CH4, CO2, H2, and H2S has escalated the biogas utilization from only a fuel into a building block of higher fuel quality, aqua plantation, or even for biochemicals production (Buono et al., 2014). A pure CO2/CH4 mixture can be upgraded to syngas [carbon monoxide (CO) and H2] by catalytic reforming. The CO and H2 gases can be upgraded to liquid fuels (long-chain hydrocarbons) by the Fischer-Tropsch synthesis process using metals and metal carbides (iron, cobalt, and ruthenium) as a catalyst (Jahangiri et al., 2014). An alternative utilization of syngas is H2 production by the water-gas-shift reaction that converts CO and H2O to CO2 and H2, since H2 is an ideal energy source due to its zero GHG emissions (Buono et al., 2014). An alternative utilization of CH4 is to produce ethylene, one of the most valuable raw materials in the petrochemical and plastic industries, via the one-step oxidative coupling of CH4. The upgrading of CH4 to ethylene promotes the valorization of biogas to value-added products (Jahangiri et al., 2014). This process is promising at an industrial scale because of its high CH4 conversion level (97%) and ethylene selectivity of up to 88% (Ryckebosch et al., 2011). The obtained ethylene can be used to produce polyethylene, one of the common polymeric thermoplastic materials used nowadays, through the polymerization reaction of ethylene monomers with Zigler-Natta catalyst. Ethylene is also a building block for the production of thermoplastic elastomer, polyethylene resin, block copolymer, and synthetic rubber (ethylene-propylene diene rubber) (Ryckebosch et al., 2011). Figure 4 shows the overview of biogas production and utilization.
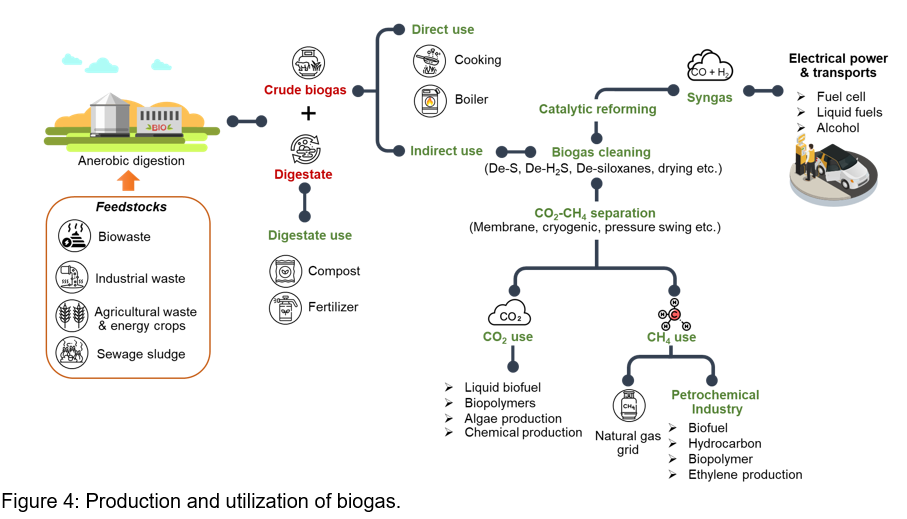
CONCLUSION
The success in promoting the biogas system in Thailand in the past focused on waste treatment coupled with energy generation. The biogas production chain can have many advantages (for example, reducing costs and increasing GHG savings). Thailand is currently moving towards the BCG concept of not only waste treatment but also value-added production creation. The circular economy concept implementation in Thai cassava starch industry depends on the key drivers of technology, economic, regulatory, and social responsibility. The biogas production technology in Thailand is mature. The technology aiming to utilize solid wastes, which has slow digestibility, are required. The income from selling electricity to the grid have attracted the investment on the biogas system. Such business models are in need to overcome the high investment cost. Thus, the supporting policy particularly on the tax-incentive and financial subsidization is necessary. While the regulatory, in term of the GHG emission reduction and wastewater discharge laws have driven the biogas technology adoption, increasing the market demand on biogas system. The last driver is social responsibility. It can be promoted by the regulation prohibiting any action contributing to negative social impact. Dissemination of the knowledge to educate community needs to be performed. These are lesson learned on behalf of Thailand, ASEAN countries should continue their research, development, and technology transfer efforts in biogas production and find a special role for biogas production, not only for waste treatment and energy generation but also for value-added product creation to achieve the circular economy concept for sustainability.
REFERENCES
Agency, I. R. E. (2017). Renewable Energy Outlook: Thailand.
Agency, N. S. a. T. D. (2018). BCG Economy. Retrieved from http://nstdachannel.tv/20181009-bcg-economy
Awe, O. W., Zhao, Y., Nzihou, A., Minh, D. P., & Lyczko, N. (2017). A Review of Biogas Utilisation, Purification and Upgrading Technologies. Waste and Biomass Valorization, 8(2), 267-283. doi:10.1007/s12649-016-9826-4
Boonpa, S., & Sharp, A. (2017). Waste-to-energy policy in Thailand. Energy Sources, Part B: Economics, Planning, and Policy, 12, 1-9. doi:10.1080/15567249.2016.1176088
Buono, S., Langellotti, A. L., Martello, A., Rinna, F., & Fogliano, V. (2014). Functional ingredients from microalgae. Food Funct, 5(8), 1669-1685. doi:10.1039/c4fo00125g
Buranasingh, N. (2020). Biogas: the product from manure to renewable energy. from The Secretariat of The House of Representatives.
C.P, F. (2020). CPF supports biogas production in animal farm and factory, for community and energy sustainability. Retrieved from https://www.cpfworldwide.com/th/media-center/sustainability-1352
company, T. E. a. E. D. (2021). The Project of Biogas Production Promotion. Retrieved from http://teed.co.th/%E0%B9%82%E0%B8%84%E0%B8%A3%E0%B8%87%E0%B8%81%E0%B8%B2...
Efficiency. (2016). Compressed biomethan gas: CBG.
Efficiency, D. o. A. E. D. a. (2021a). Energy Situation of Thailand 2020 Report. Retrieved from www.dede.go.th/ ewt_news.php?nid=47349
Efficiency, D. o. A. E. D. a. (2021b). Various Types of Biogas Production. Retrieved from http://webkc.dede.go.th/testmax/node/184
Environment, T. J. G. S. o. E. a. (2014). ASEAN Bioenergy Technology Status Report. Retrieved from http://www.sti.or.th/th/images/stories/files/asean%20bioenergy%2036pages...
Hwang, I. Y., Nguyen, A. D., Nguyen, T. T., Nguyen, L. T., Lee, O. K., & Lee, E. Y. (2018). Biological conversion of methane to chemicals and fuels: technical challenges and issues. Appl Microbiol Biotechnol, 102(7), 3071-3080. doi:10.1007/s00253-018-8842-7
Intarangsi, A., Kiatpakdee, W. (2000). Re: The Experience on Biogas Technology in Thailand: From Development to Delivery.
ITDG, I. C., IT Power and ITDG Latin America. (2007). Sustainable energy for poverty reduction: an action plan. Retrieved from https://www.greenpeace.org.cn/china/Global/china/_planet-2/report/2007/1...
Jahangiri, H., Bennett, J., Mahjoubi, P., Wilson, K., & Gu, S. (2014). A review of advanced catalyst development for Fischer–Tropsch synthesis of hydrocarbons from biomass derived syn-gas. Catalysis Science & Technology, 4(8), 2210-2229. doi:10.1039/C4CY00327F
Kadam, R., & Panwar, N. L. (2017). Recent advancement in biogas enrichment and its applications. Renewable and Sustainable Energy Reviews, 73, 892-903. doi:https://doi.org/10.1016/j.rser.2017.01.167
Krenek, A. (2020). How to implement a WTO-compatible full border carbon adjustment as an important part of the European Green Deal. Retrieved from https://www.oegfe.at/policy-briefs/wto-compatible-bca-green-deal/?lang=e...
Lerdlattaporn, R. P., Chantaraporn; Trakulvichean, Sivalee; Songkasiri, Warinthorn. (2021). Implementing circular economy concept by converting cassava pulp and wastewater to biogas for sustainable production in starch industry. Sustainable Environment Research, 31(1), 20. doi:10.1186/s42834-021-00093-9
Office, E. P. a. P. (2020). Energy Statistics of Thailand 2020.
Omer, A. M. (2005). The energy potential of biomass and prospects for the future in the Sudan. Overview of renewable and sustainable energy sources, 9(1), 1-27.
Paolini, V., Petracchini, F., Segreto, M., Tomassetti, L., Naja, N., & Cecinato, A. (2018). Environmental impact of biogas: A short review of current knowledge. J Environ Sci Health A Tox Hazard Subst Environ Eng, 53(10), 899-906. doi:10.1080/10934529.2018.1459076
Ryckebosch, E., Drouillon, M., & Vervaeren, H. (2011). Techniques for transformation of biogas to biomethane. Biomass and Bioenergy, 35(5), 1633-1645. doi:https://doi.org/10.1016/j.biombioe.2011.02.033
Siteur, J. (2012). Rapid deployment of industrial biogas in Thailand: Factors of success. Paper presented at the ECEEE 2012 SUMMER STUDY on Energy efficiency in industry, Papendal Hotel and Conference Centre, Arnhem, The Netherlands.
Suwanasri, K., Trakulvichean, S., Grudloyma, U., Songkasiri, W., Commins, T., Chaiprasert, P., & Tanticharoen, M. (2015). Biogas – Key Success Factors for Promotion in Thailand. Journal of Sustainable Energy and Environment, 25-30.
Tonrangklang, P., Therdyothin, A., & Preechawuttipong, I. (2017). Overview of Biogas Production Potential from Industry Sector to Produce Compressed Bio-methane Gas in Thailand. Energy Procedia, 138, 919-924. doi:https://doi.org/10.1016/j.egypro.2017.10.140
Trakulvichean, S. C., P.; Otmakhova, J.; Songkasiri, W. (2017). Comparison of fermented animal feed and mushroom growth media as two value-added options for waste Cassava pulp management. Waste Management & Research, 35, 1210 - 1219.
Trakulvichean, S. C., Pawinee; Otmakhova, Julia; Songkasiri, Warinthorn. (2019). Integrated Economic and Environmental Assessment of Biogas and Bioethanol Production from Cassava Cellulosic Waste. Waste and Biomass Valorization, 10. doi:10.1007/s12649-017-0076-x
Wang, S., Liu, Q., Li, J., & Wang, Z. (2021). Methane in wastewater treatment plants: status, characteristics, and bioconversion feasibility by methane oxidizing bacteria for high value-added chemicals production and wastewater treatment. Water Research, 198, 117122. doi:https://doi.org/10.1016/j.watres.2021.117122
Yentekakis, I., & Goula, G. (2017). Biogas Management: Advanced Utilization for Production of Renewable Energy and Added-value Chemicals. Frontiers in Environmental Science, 5.
Yousef, A. M. I., Eldrainy, Y. A., El-Maghlany, W. M., & Attia, A. (2016). Upgrading biogas by a low-temperature CO2 removal technique. Alexandria Engineering Journal, 55(2), 1143-1150. doi:https://doi.org/10.1016/j.aej.2016.03.026
Biogas in Thailand: an Emerging Industry from Agricultural By-products
ABSTRACT
Biogas, which is comprised of methane and carbon dioxide, is a renewable energy source that is produced through anaerobic digestion by a consortium of anaerobic microorganisms. Various organic materials, including organic wastes, organic fractions of municipal solid wastes, wastewaters from agro-industries, and energy crops, can be utilized as a substrate for biogas production. Biogas helps a community or a country to move towards energy security, since it can be used to replace heat or to generate electricity. Many developed countries, such as Germany, have successfully implemented an integrated renewable energy platform that includes biogas and other renewable energy resources, e.g. wind and solar, both for environmental benefits and economic revenues. Electricity from renewable energy sources has become a commodity. On another note, renewable energy markets have not been widely achieved in developing countries. Most countries still focus on waste treatment and utilization due to several barriers. In some cases, government policy is the main barrier to the promotion of renewable energy usage. This paper reviews the current status, promotion policy, and limiting barriers obstructing the opportunity for biogas utilization as a commodity. We hope that in the near future biogas, as a renewable energy source and as an originated component for other value-added products, will become fully exploited.
Keywords: Biogas, energy security, environmental benefit, waste treatment, renewable energy
INTRODUCTION
Thailand has relied on high levels of imported energy, at approximately 50% of all its energy consumption and increasing since the mid-1980s (Environment, 2014). In 2020, the total energy consumption in Thailand was equal to 76,928 ktoe, of which 15.1% was derived from renewable energy (RE) (D. o. A. E. D. a. Efficiency, 2021). The major RE consumption is in the form of heat, but the share of electricity and biofuels as RE is growing. The Alternative Energy Development Plan was first implemented in 2012 with the aim of increasing the proportion of alternative energy consumption to up to 30% of the total energy consumption (adjusted in 2019). Further, Thailand’s 20-year National Policy Plan (2017–2036) focuses on bio-economy, circular economy, and green economy (BCG Economy). This concept can solve natural resource problems and improve the environment and social sustainability. The BCG economy for Thailand will focus on using raw materials more efficiently, minimizing waste, improving production efficiency, upgrading technology, and creating innovation for higher efficiency. Biogas, a renewable energy source, can be one BCG solution for the renewable energy promotion scheme as well as an alternative for reducing waste and environmental impacts, subsequently reducing greenhouse gas (GHG) emissions (N. S. a. T. D. Agency, 2018).
Biogas is a naturally-occurring biological process, in which anaerobic microorganisms transform organic matters to methane (CH4) and carbon dioxide (CO2) in the absence of oxygen. The advantages of an anaerobic process for organic waste treatment are that it requires less energy and less space for building a treatment system than that of an aerobic process. Biogas systems have been utilized as waste and wastewater treatment units in various sectors: agriculture, agro- and food industries, and even the energy-producing sector. Raw materials for biogas generation are agricultural residues, wastes from animal farms, wastewater from agro- and food industries, or the organic fraction of municipal solid wastes.
A typical biogas system has three main components: raw material preliminary unit, biogas production unit, and post-treatment units (Figure 1). The objectives of the preliminary or pre-treatment unit are raw material size reduction, removal of inorganic materials, and toxicity neutralization. Pretreatment technologies are mechanical, thermal, enzymatic, and chemical treatments.
A biogas reactor, or biogas producing unit, can be classified in several ways, based on the system efficiency, the microbial structure inside the reactor, the operating temperature, or the amount of solids entering the system. The biogas system based on the efficiency can be divided into low-rate, conventional rate and high-rate systems, which are classified by the organic loading rate of the system (<2, 2-4, >4 kg of COD/m3 of reactor/day, respectively), where COD stands for chemical oxygen demand, indicating the amount of organic matter. There are two types of microbial structure inside the reactor: suspended growth and attached growth. The reactor with the suspended growth type is where anaerobic microbial consortium forms anaerobic granules suspending inside the reactor, while, with the attached growth type, the anaerobic microbial consortium forms a biofilm, which attaches onto the media inside the reactor. The two operating temperatures are mesophilic (approximately 35oC) and thermophilic (approximately 55oC). The solids entering the reactor influence the design of the system. A typical reactor that has the influent of <20% of the solid content called a wet system, while a reactor with >20% of solid content is called a dry system. Biogas technology is at a fully commercialized level (Suwanasri et al., 2015). Most biogas systems require post-treatment for all three phases of the effluent – secondary treatment open ponds for the wastewater, biogas cleaning for the biogas, and sludge treatment or utilization for the solid waste. Most biogas cleaning technologies to remove contaminants, such as hydrogen sulfide (H2S), are at fully commercialized levels. In general, the utilization of biogas in Thailand is in the form of heat, electricity, vehicular fuel, and combined heat and power, and all of which are already in fully commercialized levels (Suwanasri et al., 2015).
BIOGAS AS WASTE TREATMENT
Implementation of the biogas system for waste and wastewater treatment options has been initiated in Thailand since the early `80s. The biogas system was first promoted in animal farms as a waste treatment unit. Unfortunately, it was unsuccessful due to a lack of understanding and construction standards leading to the system failure. To overcome these drawbacks, the Thai government created initiatives in collaboration with the German Technical Corporation, which is now known as the German International Corporation for the promotion of the biogas systems in animal farms. Universities collaborated with the Department of Agricultural Extension, Ministry of Agriculture, to disseminate knowledge on the Thai biogas technology for the treatment of wastewater from animal farms. Further, in the 2000s, the Ministry of Energy promoted the use of biogas systems in agro-industries utilizing local technology from universities and private companies.
One success case of biogas technology transfer in Thailand is the transfer of high-rate anaerobic fixed film (AFF) technology from King Mongkut’s University of Technology Thonburi and the National Center for Genetic Engineering and Biotechnology to agro- and food industries, especially cassava starch, palm oil, and food factories (shown in Figure 2). Media installation in the AFF helps preventing sludge wash-out, as shown in Figure 3(a). The AFF has high microorganism concentration, consequently high system efficiency and resistant to high-concentration wastewater. Each biogas system was designed based upon the type of wastes and wastewaters, the amount of waste fed into the system, and the selected technology. Factories selected the biogas utilization option based upon and customized to their needs, receiving economic benefit along with fulfilling their waste treatment obligation. Table 1 presents the biogas utilization alternatives with an estimated economic impact in each type of industry using this high-rate technology.
Another biogas technology adoption can be seen at the Charoen Pokphand Foods (CPF). The CPF implemented the conventional-rate anaerobic baffled reactor (ABR) technology for treating wastewater from animal farms. Baffles inside the ABR control direction of fluid flow, make it possible to extend the sludge retention time, as shown in Figure 3(b). The project costs approximately USD 800,000, which the Energy Policy and Planning Office, Ministry of Energy, partially subsidized (Buranasingh, 2020). The ABR helped eliminate the smell of the poultry manure, which was of concern to the nearby community. On the other hand, it generated biogas at 2,500 m3/d, which was used for producing heat and electricity, replacing 360,000 L/y of fuel oil. In 2019, the CPF and its affiliated companies produced 48.61 million-m3 of biogas, which saved a production cost of USD 7.8 million and reduced the GHG emission by 33,268 ton-CO2eq (C.P, 2020).
The other success case of the biogas implementation in the cassava starch industry was at the Korat waste to energy project (KWTE), in 2003. The project set up a business model between a biogas company and a cassava starch factory, as the Built-Own-Operate-Transfer (BOOT). The biogas company invested in biogas production along with electricity production, while the cassava starch factory provided land, wastewater, and the commitment to buy the electricity from the biogas company. Under the BOOT agreement, the electricity price was set to be 20% lower than the grid. In the first year of operation, the biogas plant supplied the energy replacing the 7.5 million L of fuel oil and 35 GWh of grid’s electricity, which was almost self-sufficient for the cassava starch factory. The KWTE overperformed with a high profitability, at the IRR of 15–17%, along with promoting an environmentally-friendly image to the cassava starch factory (Siteur, 2012). This success case attracted other companies to adopt the biogas production technology. Between 2004 and 2011, biogas technology installation in the Thai-cassava starch factories increased significantly. At the end of 2011, almost 80% of 77 Thai-cassava starch factories had installed biogas plants.
According to the calculations of the model for the production of biogas from cassava starch waste within ten years, the achievement of commercial benefits demonstrates a promising nature for investment in such enterprises by state corporations and financing through state programs to support climate and energy policy. Cellulosic material is a high potential raw material for the 2nd generation biogas production, in Thailand. Thai cassava starch industry also generates cassava pulp, as a solid residue of cassava root remained after starch extraction, of 12.5 million tons annually (Trakulvichean et al., 2017). With the circularity concept, several approaches have been attempted to utilize the cassava pulp for both agriculture- (animal feed, mushroom growth media, fertilizer) and renewable energy-sector (bioethanol, biogas, and refused derived fuel (RDF)). Producing the electricity from the cassava pulp through the biogas production technology is economically feasible (Lerdlattaporn et al., 2021). The investment on such technology depends on biogas utilization option, which is so far aimed mainly as heat source for the starch production process for producing the electricity. While the latter option is limited by the capability of the grid to buy-back the electricity and still needing the policy support on the electricity pricing mechanism. By-products from the cassava starch production are utilized under the circular economy concept. All end products, e.g., treated wastewater, sand, soil, peel, and cassava pulp can be used for the cassava root cultivation. So far, several factories import the technology but there are still lack of knowledge to utilize the technology. Which mislead technology feasibility to be questioned among the industries. These pulp utilization options need to be balanced with selling the pulp as animal feed to maximize the economic benefits for private companies. Then, the renewable energy production from the cassava pulp is needing the determination of the priorities for new cassava pulp markets, supportive policies, and a roadmap for achieving these priorities are also needed. Government initiatives may motivate cassava starch factories to choose beneficial options for the sustainable waste management of pulp. Biogas production from cassava pulp is still in need of government support to demonstrate its local proven biogas technologies are similar to biogas production from cassava starch wastewater (Trakulvichean et al., 2019).
POLICY ON BIOGAS PROMOTION IN THAILAND: PAST TO CURRENT STATE
In the `70s, the Sanitary Division under the Ministry of Public Health of Thailand began to invest in small-sized biogas plants for resolving a sanitary issue. As aforementioned, in the late 1980s, the oil price increased drastically worldwide, and so the Royal Thai government started to push biogas as an alternative energy source, which caused the major movement in developing an incentive policy for biogas plant investment (Intarangsi & Kiatpakdee, 2000). Initially, the investment cost in biogas, though effective productivity-wise, was too high to be attractive for the private sector (Suwanasri et al., 2015); therefore, the early incentive policy focused on increasing the financial competitiveness of biogas as an alternative power source. This was achieved through the measure of electricity pricing adder in the feed-in tariff system, the financial support from the energy conservation promotion fund, the investment promotion by the Thailand Board of Investment, and the promotion measure of technology on biogas production for livestock and other relevant enterprises. Different promotion schemes were locally implemented and were focused on both the promotion of the governmental sector and the private consulting firms for biogas production. For example, some projects have been implemented from those private consultants performed by the Thai Energy and Environment Development company and the projects from the governmental sector were supported by the Department of Alternative Energy Development and Efficiency (company, 2021; D. o. A. E. D. a. Efficiency, 2021b). From 2012, the national energy policy and the Alternative Energy Development Plan were implemented to increase the proportion of alternative energy consumption up to 30% of the total energy consumption, which became the second wave of the promotional policy for biogas.
The big difference in the recent incentive program was that the recent policy aimed to target the waste-to-energy perspective of biogas, especially municipal solid waste treatment, more than just alternative energy due to the enormous amount of waste produced in Thailand (Boonpa & Sharp, 2017). According to the rise of the circular economy, the future of biogas in Thailand might have to readjust its position by strengthening the environmentally friendly factors. According to the EU net-zero emission goals in 2050, the draft of the European Green Deal has been pushed to take a major effect in the supply chain of the EU in the commodities products, especially food sectors. Carbon Tax Adjustment and Tax Credit will be applied in order to justify the cost to go greener in food production, which causes producers who want to sell their products at a compatible price in the EU to seek greener energy sources to lower their carbon footprint (Krenek, 2020). In Thailand, from 2023 the Securities and Exchange Commission will highlight the awareness of alternative energy usage of a company by forcing the company to report its current GHG emission, current carbon footprint, and roadmap to go greener in the report for the annual general meeting of shareholders (SEC, 2021). These top-down enforcers will help push the members of the private sector in Thailand to make a higher proportion of their energy consumption come from alternative renewable energy sources, in which biogas has approximately half of the stake in Thailand (I. R. E. Agency, 2017; Office, 2020).
BIOGAS AS A COMMODITY IN THE GLOBAL ENERGY MARKET
The lack of affordable energy services is closely linked to many sustainable development issues, such as poverty reduction, the advancement of women, environmental protection, and job creation. The emphasis on institution-building and the expansion of political dialogue is necessary to create a social, economic, and political enabling environment to transition to a more sustainable future. On the other hand, technologies for generating energy from biomass are a promising option that will significantly impact developing countries where the current level of energy services is low (Omer, 2005).
Biogas has many advantages and is used for various purposes, such as electricity production, thermal energy production, and use as a transport fuel. Many countries have chosen the use of renewable energy sources as one of the priorities of their national policy, including the EU countries, the USA, Brazil, Canada, Japan, China, and others. In Europe, Germany is the leader in terms of the amount of biogas produced, while Denmark is the leader in terms of the intensity of biogas usage (this type of fuel provides almost 20% of the country's energy consumption). The policy elevated the number of biogas plants using energy crops as well as wastes from animal farms and factories to generate electricity, which can be sold as a commodity in an energy market. Further, within the Green Course program framework, the European Commission sets the task of building a climate-neutral European economy by 2050. One of the primary means of achieving it is the complete replacement of natural gas with carbon-free and renewable analogs: biogas, biomethanol, and hydrogen (H2).
The United Nations (UN) Commission on Sustainable Development (CSD) identified access to basic energy services as an essential element of sustainable development. According to the CSD, to implement the goal accepted by the international community to halve the proportion of people living on less than USD 1 per day by 2015, access to affordable energy services is a prerequisite (ITDG, 2007). There is a strong correlation between energy availability and education, health, urban migration, empowerment, local employment and income generation, and the overall quality of life.
The change in the global energy supply requires new solutions in the agro- and food industries, where biogas can become one of the drivers of economic growth in Southeast Asia. When making strategic decisions on creating enterprises for the production of biogas, it is important to take into account the economic benefits and the impact on the environment, socio-economic, and budgetary efficiency. The economic efficiency of biogas plants is especially high when processing a large continuous stream of wastes. In addition, biogas energy is also a source of cheap complex organic fertilizers, which are formed as a by-product in biogas production. At the same time, it is advisable to consider the production of biogas as the final link in the production chain for the disposal of production waste and the transformation of waste into a commodity with high added value. From the point of view of the producers of export goods from agro- and food raw materials, the possibility of creating a private-public partnership will allow the enterprise to monetize the costs associated with waste losses. From the state's point of view, an increase in revenues to the state budget is obtained by increasing the profits from export production and obtaining a share of electricity that can close or reduce the required level of electricity imports or to reduce the price of electricity due to income from biogas.
BIOGAS AS ORIGINATED COMPOUND FOR VALUE-ADDED PRODUCTS
Direct use of biogas (CH4 as natural gas, CO2 as gas or liquid use in greenhouse or industry)
By volume, biogas contains 50–70% of CH4, 30–50% of CO2, and small amounts of H2 and other contaminants, such water, siloxanes, volatile organic compounds, and H2S (Awe et al., 2017; Paolini et al., 2018; Tonrangklang et al., 2017). The proportion of CH4 in the biogas affects the heating value of the biogas, whereas CO2 does not provide heat (Yousef et al., 2016). A normal cubic meter of CH4 contains 10 kWh of energy (Yentekakis & Goula, 2017), which is close to that of natural gas (energy content of 11 kWh). Over the past decade, biogas has been upgraded to biomethane for injecting into the natural gas grid and even as compressed biomethane gas (CBG) as a fuel in the transportation sector (Efficiency, 2016).
In Thailand, the first pilot plant producing CBG, with a production capacity of 8 kg/h, occurred in 2012 (Wang et al., 2021). Permission and quality guarantee of CH4 gas are regulated before injection into the grid (Kadam & Panwar, 2017). In recent years, worldwide biogas utilization has not only focused on fuel but also its use as a raw material for biochemicals production (Hwang et al., 2018; Wang et al., 2021). Using biogas purification technologies, such as pressure swing absorption, CO2 is separated and can be delivered to various destinations. In the Netherlands and Belgium, CO2 is delivered to greenhouses for crop and flower production or it can be directly supplied to algae production, which is an energy crop and functional food. Trends of biogas utilization in Thailand are shifting in the same manner.
Transformation of biogas to other products: CH4, CO2, and H2
Purification of the CH4, CO2, H2, and H2S has escalated the biogas utilization from only a fuel into a building block of higher fuel quality, aqua plantation, or even for biochemicals production (Buono et al., 2014). A pure CO2/CH4 mixture can be upgraded to syngas [carbon monoxide (CO) and H2] by catalytic reforming. The CO and H2 gases can be upgraded to liquid fuels (long-chain hydrocarbons) by the Fischer-Tropsch synthesis process using metals and metal carbides (iron, cobalt, and ruthenium) as a catalyst (Jahangiri et al., 2014). An alternative utilization of syngas is H2 production by the water-gas-shift reaction that converts CO and H2O to CO2 and H2, since H2 is an ideal energy source due to its zero GHG emissions (Buono et al., 2014). An alternative utilization of CH4 is to produce ethylene, one of the most valuable raw materials in the petrochemical and plastic industries, via the one-step oxidative coupling of CH4. The upgrading of CH4 to ethylene promotes the valorization of biogas to value-added products (Jahangiri et al., 2014). This process is promising at an industrial scale because of its high CH4 conversion level (97%) and ethylene selectivity of up to 88% (Ryckebosch et al., 2011). The obtained ethylene can be used to produce polyethylene, one of the common polymeric thermoplastic materials used nowadays, through the polymerization reaction of ethylene monomers with Zigler-Natta catalyst. Ethylene is also a building block for the production of thermoplastic elastomer, polyethylene resin, block copolymer, and synthetic rubber (ethylene-propylene diene rubber) (Ryckebosch et al., 2011). Figure 4 shows the overview of biogas production and utilization.
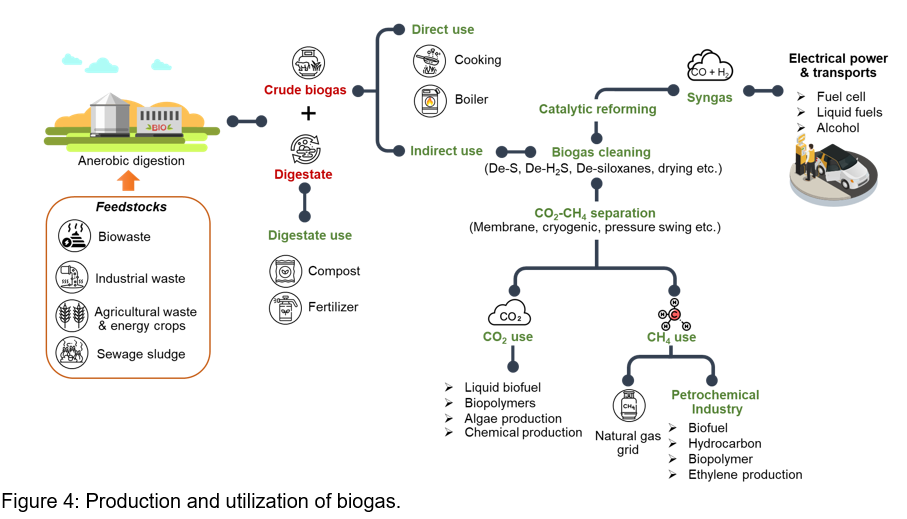
CONCLUSION
The success in promoting the biogas system in Thailand in the past focused on waste treatment coupled with energy generation. The biogas production chain can have many advantages (for example, reducing costs and increasing GHG savings). Thailand is currently moving towards the BCG concept of not only waste treatment but also value-added production creation. The circular economy concept implementation in Thai cassava starch industry depends on the key drivers of technology, economic, regulatory, and social responsibility. The biogas production technology in Thailand is mature. The technology aiming to utilize solid wastes, which has slow digestibility, are required. The income from selling electricity to the grid have attracted the investment on the biogas system. Such business models are in need to overcome the high investment cost. Thus, the supporting policy particularly on the tax-incentive and financial subsidization is necessary. While the regulatory, in term of the GHG emission reduction and wastewater discharge laws have driven the biogas technology adoption, increasing the market demand on biogas system. The last driver is social responsibility. It can be promoted by the regulation prohibiting any action contributing to negative social impact. Dissemination of the knowledge to educate community needs to be performed. These are lesson learned on behalf of Thailand, ASEAN countries should continue their research, development, and technology transfer efforts in biogas production and find a special role for biogas production, not only for waste treatment and energy generation but also for value-added product creation to achieve the circular economy concept for sustainability.
REFERENCES
Agency, I. R. E. (2017). Renewable Energy Outlook: Thailand.
Agency, N. S. a. T. D. (2018). BCG Economy. Retrieved from http://nstdachannel.tv/20181009-bcg-economy
Awe, O. W., Zhao, Y., Nzihou, A., Minh, D. P., & Lyczko, N. (2017). A Review of Biogas Utilisation, Purification and Upgrading Technologies. Waste and Biomass Valorization, 8(2), 267-283. doi:10.1007/s12649-016-9826-4
Boonpa, S., & Sharp, A. (2017). Waste-to-energy policy in Thailand. Energy Sources, Part B: Economics, Planning, and Policy, 12, 1-9. doi:10.1080/15567249.2016.1176088
Buono, S., Langellotti, A. L., Martello, A., Rinna, F., & Fogliano, V. (2014). Functional ingredients from microalgae. Food Funct, 5(8), 1669-1685. doi:10.1039/c4fo00125g
Buranasingh, N. (2020). Biogas: the product from manure to renewable energy. from The Secretariat of The House of Representatives.
C.P, F. (2020). CPF supports biogas production in animal farm and factory, for community and energy sustainability. Retrieved from https://www.cpfworldwide.com/th/media-center/sustainability-1352
company, T. E. a. E. D. (2021). The Project of Biogas Production Promotion. Retrieved from http://teed.co.th/%E0%B9%82%E0%B8%84%E0%B8%A3%E0%B8%87%E0%B8%81%E0%B8%B2...
Efficiency. (2016). Compressed biomethan gas: CBG.
Efficiency, D. o. A. E. D. a. (2021a). Energy Situation of Thailand 2020 Report. Retrieved from www.dede.go.th/ ewt_news.php?nid=47349
Efficiency, D. o. A. E. D. a. (2021b). Various Types of Biogas Production. Retrieved from http://webkc.dede.go.th/testmax/node/184
Environment, T. J. G. S. o. E. a. (2014). ASEAN Bioenergy Technology Status Report. Retrieved from http://www.sti.or.th/th/images/stories/files/asean%20bioenergy%2036pages...
Hwang, I. Y., Nguyen, A. D., Nguyen, T. T., Nguyen, L. T., Lee, O. K., & Lee, E. Y. (2018). Biological conversion of methane to chemicals and fuels: technical challenges and issues. Appl Microbiol Biotechnol, 102(7), 3071-3080. doi:10.1007/s00253-018-8842-7
Intarangsi, A., Kiatpakdee, W. (2000). Re: The Experience on Biogas Technology in Thailand: From Development to Delivery.
ITDG, I. C., IT Power and ITDG Latin America. (2007). Sustainable energy for poverty reduction: an action plan. Retrieved from https://www.greenpeace.org.cn/china/Global/china/_planet-2/report/2007/1...
Jahangiri, H., Bennett, J., Mahjoubi, P., Wilson, K., & Gu, S. (2014). A review of advanced catalyst development for Fischer–Tropsch synthesis of hydrocarbons from biomass derived syn-gas. Catalysis Science & Technology, 4(8), 2210-2229. doi:10.1039/C4CY00327F
Kadam, R., & Panwar, N. L. (2017). Recent advancement in biogas enrichment and its applications. Renewable and Sustainable Energy Reviews, 73, 892-903. doi:https://doi.org/10.1016/j.rser.2017.01.167
Krenek, A. (2020). How to implement a WTO-compatible full border carbon adjustment as an important part of the European Green Deal. Retrieved from https://www.oegfe.at/policy-briefs/wto-compatible-bca-green-deal/?lang=e...
Lerdlattaporn, R. P., Chantaraporn; Trakulvichean, Sivalee; Songkasiri, Warinthorn. (2021). Implementing circular economy concept by converting cassava pulp and wastewater to biogas for sustainable production in starch industry. Sustainable Environment Research, 31(1), 20. doi:10.1186/s42834-021-00093-9
Office, E. P. a. P. (2020). Energy Statistics of Thailand 2020.
Omer, A. M. (2005). The energy potential of biomass and prospects for the future in the Sudan. Overview of renewable and sustainable energy sources, 9(1), 1-27.
Paolini, V., Petracchini, F., Segreto, M., Tomassetti, L., Naja, N., & Cecinato, A. (2018). Environmental impact of biogas: A short review of current knowledge. J Environ Sci Health A Tox Hazard Subst Environ Eng, 53(10), 899-906. doi:10.1080/10934529.2018.1459076
Ryckebosch, E., Drouillon, M., & Vervaeren, H. (2011). Techniques for transformation of biogas to biomethane. Biomass and Bioenergy, 35(5), 1633-1645. doi:https://doi.org/10.1016/j.biombioe.2011.02.033
Siteur, J. (2012). Rapid deployment of industrial biogas in Thailand: Factors of success. Paper presented at the ECEEE 2012 SUMMER STUDY on Energy efficiency in industry, Papendal Hotel and Conference Centre, Arnhem, The Netherlands.
Suwanasri, K., Trakulvichean, S., Grudloyma, U., Songkasiri, W., Commins, T., Chaiprasert, P., & Tanticharoen, M. (2015). Biogas – Key Success Factors for Promotion in Thailand. Journal of Sustainable Energy and Environment, 25-30.
Tonrangklang, P., Therdyothin, A., & Preechawuttipong, I. (2017). Overview of Biogas Production Potential from Industry Sector to Produce Compressed Bio-methane Gas in Thailand. Energy Procedia, 138, 919-924. doi:https://doi.org/10.1016/j.egypro.2017.10.140
Trakulvichean, S. C., P.; Otmakhova, J.; Songkasiri, W. (2017). Comparison of fermented animal feed and mushroom growth media as two value-added options for waste Cassava pulp management. Waste Management & Research, 35, 1210 - 1219.
Trakulvichean, S. C., Pawinee; Otmakhova, Julia; Songkasiri, Warinthorn. (2019). Integrated Economic and Environmental Assessment of Biogas and Bioethanol Production from Cassava Cellulosic Waste. Waste and Biomass Valorization, 10. doi:10.1007/s12649-017-0076-x
Wang, S., Liu, Q., Li, J., & Wang, Z. (2021). Methane in wastewater treatment plants: status, characteristics, and bioconversion feasibility by methane oxidizing bacteria for high value-added chemicals production and wastewater treatment. Water Research, 198, 117122. doi:https://doi.org/10.1016/j.watres.2021.117122
Yentekakis, I., & Goula, G. (2017). Biogas Management: Advanced Utilization for Production of Renewable Energy and Added-value Chemicals. Frontiers in Environmental Science, 5.
Yousef, A. M. I., Eldrainy, Y. A., El-Maghlany, W. M., & Attia, A. (2016). Upgrading biogas by a low-temperature CO2 removal technique. Alexandria Engineering Journal, 55(2), 1143-1150. doi:https://doi.org/10.1016/j.aej.2016.03.026